Prof. Dr. John Douglas Cockcroft > Research Profile
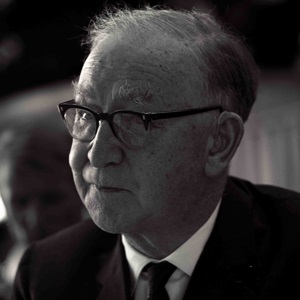
by Luisa Bonolis
John Douglas Cockcroft
Nobel Prize in Physics 1951 together with Ernest T.S. Walton
"for their pioneer work on the transmutation of atomic nuclei by artificially accelerated atomic particles".
Becoming Rutherford's student in Cambridge
John Cockcroft was born on 27 May 1897 and spent his early years in Todmorden, on the borders of Lancashire and Yorkshire, where his family had lived for centuries. When he was at secondary school in the early 1910s, he became especially interested in atomic physics through the popular accounts of the pioneer work of J. J. Thomson and Ernest Rutherford, who by that time were both Nobel Laureates. Thomson had been awarded the Nobel Prize in Physics 1906 for his theoretical and experimental investigations on the conduction of electricity by gases, which had eventually led to the discovery of the electron, while Rutherford, who had just postulated the concept of atomic nucleus, had received the 1908 Nobel Prize in Chemistry for his pioneering investigations in the field of radioactivity and the chemistry of radioactive substances.
Inspired by these fascinating novelties, Cockcroft developed an ambition to do research work in experimental physics, but was advised to study mathematics first. In the autumn of 1914 he matriculated at Manchester University where he was fortunate enough to be able to attend some first-year lectures given by Rutherford. With the advent of World War I, Cockcroft joined the British Armed Forces in 1915 and served in the Royal Field Artillery. At the end of the war he returned to Manchester to study electrical engineering at the College of Technology, after which he worked as an engineer for the Metropolitan-Vickers Electrical Company. Soon his deep interest in mathematics and the physical sciences drew him back to the university. Like Paul Dirac, Cockcroft left engineering to study mathematics at St. John's College, Cambridge, where he took the Mathematical Tripos in 1924. During his studies he had been encouraged by Rutherford, who had recently become the director of the Cavendish Laboratory, to spend as much time as he could in the advanced practical class in physics, where Cockcroft actually was able to learn how to conduct experiments. His wide-ranging education, a diverse and formidable combination of training in mathematics, physics and engineering, plus practical experience with the Metropolitan Vickers Electrical Company, primed Cockcroft for his future success. He was accepted by Rutherford as a research student in the Cavendish Laboratory, where frequent colloquia and seminars were of the highest standard and invited speakers came from all over the international world of physics.
Artificial disintegration of the elements and early particle accelerators
Cockcroft selected for his PhD thesis the properties of thin films of metallic vapours deposited on polished, outgassed surfaces, cooled to liquid-air temperatures. He was able to use the best vacuum technique then available in the Cavendish, which afforded him valuable experience in a technology that was to be crucial in his later work. Rutherford thought that Cockcroft's background in electrical engineering cold be useful and asked him to work with the Russian physicist Peter Kapitza, who was in charge of the magnetic laboratory, to build the equipment to be used for generating intense magnetic fields with the aim to ascertain if, and how, they might modify electron orbits and movements in atoms arranged in a well defined atomic lattice in a crystal of a metal. By this time Cockcroft had acquired a deep insight into certain aspects of electrical engineering and commanded some powerful theoretical techniques; he was thus able to design extremely efficient magnet coils in which a field of 300000 gauss was reached, the highest artificial field ever made up to that date. At that time he also designed an electromagnet for alpha-ray spectroscopy for Rutherford and a permanent magnet for beta-ray spectroscopy. He finally took his PhD in September 1928.
By 1928 Rutherford's reputation as a founding father of nuclear physics was firmly established. He had just been made president of the Royal Society, and in his presidential address he said: “I have long hoped for a source of positive particles more energetic than those emitted from natural radioactive substances.”It was in 1919 that Rutherford had achieved the first nuclear reaction by bombarding nitrogen nuclei with alpha particles from a radioactive source, the first artificial transmutation of an element.
In the following four years he and Chadwick succeeded in disintegrating several other elements. Up to that time, the greater part of information on the structure of the nucleus had come from experiments with alpha particles, but this procedure was not too promising for large-scale investigations.
Since the nucleus and the bombarding projectile (alpha particle, proton) are both positively charged and repel each other, the problem is essentially that of the penetration of potential barriers by particles. Further progress was made difficult by the limited strengths of the available radioactive sources and because the energies of the emitted positive-charged alpha particles were too low. The discovery of the neutron was still in the future, and thus charged particles had to be used to probe nuclei. It became increasingly obvious that attempts should be made to produce by artificial means suitable streams of fast particles, but the practical difficulties appeared to be immense. The potential barriers surrounding nuclei were known to be of the order of millions of electronvolts and such potentials were far beyond anything that had been applied successfully to X-ray or cathode ray tubes.
In the fall of 1927, the young Ernst Walton arrived at the Cavendish with a scholarship from Trinity College in Dublin. After Rutherford's approval, Walton began to set up an apparatus for accelerating positively charged particles, with Cockcroft helping to solve the engineering problems. In the meantime in the USA, the production of particles of sufficient energy to excite nuclear reactions was attempted almost simultaneously by different methods. Robert Van de Graaff developed a new method of generating high voltages by means of the continuous charging of a high potential spherical electrode. By 1929 a small model of what we now call the Van de Graaff Generator was built to demonstrate the soundness of the principle involved. The limitation of these types of accelerators actually arises from the maximum practical potential difference that can be held by the charged surfaces. Thereafter, larger, higher-voltage generators were made and the Van de Graaff generator came to be used throughout the world, not only as an ion accelerator for studies in low-energy nuclear physics but also for such purposes as X-ray radiography, radiation therapy, food sterilisation, and, on a limited scale, as an ion injector for the very high-energy particle accelerators.
In the same period, Ernest Lawrence, who had become Professor of Physics at Berkeley a year after his first visit to Cambridge, conceived the idea of deflecting the beam of particles into a circular orbit by means of a magnetic field and giving them a boost of energy by a small electric field twice per revolution. Although the first working model, built in 1928, which he called cyclotron, was only 10 cm in diameter and produced 80-keV protons, there appeared to be no limit, in principle, to the ultimate size of such instrument. Actually, the upper bound on energy obtainable from the cyclotron is set by relativistic effects; however, they circumvent the electrical breakdown problem existing in electrostatic accelerators and are thus still in use all over the world for nuclear science studies, radioisotope production, and medical therapy. Cyclotrons eventually proved to be the most useful for producing the highest energy particles, but the first system that successfully produced artificial nuclear reactions was built at Cavendish Laboratory.
Beginning Nuclear Physics at Cavendish Laboratory
Rutherford had used 10 MeV alpha particles from radioactive decay for his famous scattering experiments. This was considered a measure of the typical energy with which the particles in the nucleus are bound together and thus also for the Coulomb potential barrier a particle from the outside would need to cross in order to enter the nucleus. However, at this time experimental work on the energies of alpha-particles ejected from the radioactive elements had shown that these particles could have a substantially lower energy. For a time this was somewhat of a puzzle. It was a Russian theoretical physicist, the 25-year-old George Gamow, who enabled the great step forward to be taken towards "high-energy" nuclear physics. Late in 1928 Gamow visited the Cavendish. He had been working with Niels Bohr in Copenhagen, whose Institute had also hosted Werner Heisenberg, Erwin Schrödinger and Wolfgang Pauli, the young fathers of quantum mechanics who would become Nobel laureates in the field of theoretical physics. The ancient relationship between Rutherford and Bohr had also favoured a healthy cross-fertilisation between the two centres and it was thanks to a friendship between Nevill Mott of Cambridge - future Nobel Laureate in Physics in 1977 - and George Gamow, that the idea of applying the relatively new wave mechanics of those days to nuclear disintegration reached Cambridge.
By attributing wave properties to the escaping alpha particles, Gamow showed that they could simply penetrate through the potential barrier surrounding the nucleus without having a high enough energy to surmount it. In the US, Gurney and Condon had developed similar ideas in that same period. Reflecting on why Rutherford's alpha-particles had entered into the nucleus of a nitrogen atom in the 1919 experiment, Gamow applied his theory of the so-called "quantum tunnelling effect" in an inverse manner. He calculated the probability of a particle from the outside penetrating into the nucleus, even though its own energy was too small to surmount the Coulomb barrier round the nucleus. It was the first application of quantum mechanics to nuclear physics.
Cockcroft saw the manuscript and was quick to realise that tunnelling could lower the energy required for an incident positively charged particle to overcome the Coulomb barrier of a target nucleus - amounting to several million electronvolts. He immediately wrote a memorandum to Rutherford summarising his own calculations: protons with an energy of only 300 000 electronvolts should be able to penetrate into a boron nucleus. The probability was small, only about 6 in 1000, but a small current of 1 microampere provides many millions of protons each second. Rutherford was sufficiently impressed to allow Cockcroft to go ahead and suggested that Walton should collaborate with Cockcroft in the building of a positive-ion source to produce protons, and a vacuum tube to work at some hundreds of kilovolts to accelerate these protons before they struck targets of different elements. They acquired the largest induction coil in the Laboratory and built a first accelerator tube obtaining erratic beams of a few microamperes with which they bombarded elements from all parts of the periodic table. They expected to see gamma rays of very high energy, since 5-10 MeV of mass-energy should become available upon the capture of a proton by a nucleus. But they weren’t able to.
The Voltage Multiplier and the First Artificial Nuclear Disintegration
It is fortunate that, in the middle of 1931, Cockcroft and Walton had to dismantle their first apparatus, and move into a much larger room, with a high ceiling, which had been a lecture theatre. This provided the opportunity to start again from scratch, using an ingenious voltage multiplying circuit which Cockcroft had developed modifying a circuit due to the German engineer M. Schenkel. In order to obtain high steady potentials for the acceleration of protons, the basic idea was to construct a voltage quadrupling system - capable of extension to any even multiple of the transformer voltage - which used rectifiers to charge capacitors in parallel at low potential - each bearing a voltage of a few hundred volts - and discharged them in series through a load resistor to develop the high potential. The combined voltage, in this case about 700 kV, was then connected to the accelerating column in order to accelerate protons. The rectifiers were all connected in series and so could be erected as a single column and evacuated by one diffusion pump at earth potential. The proton source at the upper terminal consisted of a high-voltage discharge in hydrogen gas in a separate chamber with a small-diameter exit hole through which the hydrogen ions emerged into the accelerating tube. After acceleration down the column, the beam emerged through a channel in the grounded base-plate, where it was directed against the target.
By February 1932 Cockcroft and Walton were able to bring a narrow beam of 710 kilovolt protons out through a thin mica window in the base of the experimental tube, and to measure their range as a function of energy. And very soon the day came when they could install a target for bombardment. A light element would obviously make the most suitable target. On April 14, they set out to look for alpha particles from the disintegration of lithium. Opposite the mica window they placed the well-tried tool of Rutherford - the fluorescent screen, a piece of paper or card coated with very fine crystals of zinc sulphide, a mineral that emits small flashes of green light when the alpha-particle strike it, which can be seen with a microscope. Immediately below the accelerator tube, an observation cubicle was installed, which was covered with black velvet, so that the faint scintillations by the detector might be observed. Almost at once, at the energy of 125 kilovolts, Walton saw the bright characteristic scintillations, and a first primitive absorption experiment showed that they had a range of about 8.4 cm. A primitive coincidence experiment, carried out with two fluorescent screens, proved that the alpha particles were emitted in pairs. More refined experiments showed that the energy of the alpha particles was 8.6 million electronvolts. It was obvious then that the lithium isotope of mass 7 occasionally captured a proton and the resulting nucleus of mass 8 was being disintegrated into two alpha particles, each of mass 4 with a total energy release of about 17 million electronvolts. The combined mass of the resulting helium nuclei is actually slightly less than the combined mass of the original lithium and hydrogen nuclei. The very small change in mass becomes energy, in good agreement with the mass balance of the reaction: lithium-7 + proton -> helium-4 + helium-4 + energy. It demonstrated that the amount of energy released in this nuclear disintegration is precisely that predicted in Einstein's basic equation on the equivalence of mass and energy E=mc^2.
The first artificial nuclear disintegration brought experimental physics at the Cavendish in the 1930s out of the era of string, sealing wax and glass blowing. The results showed that nuclei could be disrupted by particles of lower energy than previously supposed.
It also was the first of its kind to excite the imagination of the public.
After World War II, the cascade rectifiers, which became known as Cockcroft-Walton generators, provided up to 4 MV and a large number of small 200-300 kV units were built for deuterium-deuterium reactions, used as sources of neutrons. They have been used since then to supply high voltages, also becoming an essential part of particle accelerators and other devices. However, only in 1951, John Cockcroft and Ernest Walton shared the Nobel Prize in Physics for the “transmutation of atomic nuclei by artificially accelerated atomic particles”. Why had it taken so long to recognise the achievement? Cockcroft and Walton as well as Lawrence were actually nominated for the years 1937-1939, but it was Ernest O. Lawrence who was awarded the Physics Nobel Prize in 1939 "for the invention and development of the cyclotron and for results obtained with it, especially with regard to artificial radioactive elements". Actually the cyclotron, which accelerated protons and deuterons at several million electronvolts and was used extensively in early investigations of nuclear reactions involving neutrons and artificial radioactivity, attracted a special attention for its growing role in the systematic production of radioisotopes used for therapeutic application on human patients. Especially John Lawrence, the physician brother of Ernest, soon demonstrated the isotope-making cyclotron's worth in disease research. It is most probable that such important social impact had a main role in the decision of 1939.
Bibliography
Cathcart, B. (2004). The Fly in the Cathedral. Penguin Books, London
Hartcup, G. and Allibone, T. E. (1984). Cockcroft and the Atom. Adam Hilger, Bristol
Oliphant, M. L. E. (1968). John Douglas Cockcroft. Biographical Memoirs of Fellows of the Royal Society 14: 139-187
Poole, M. et al. (2007). Cockcroft's subatomic legacy: splitting the atom, CERN Courier, December 2007. Nov 20, 2007, http://cerncourier.com/cws/article/cern/31864
Spence, R. (2008). Cockcroft, John Douglas. Complete Dictionary of Scientific Biography. Vol. 3. Detroit: Charles Scribner's Sons. p. 328-331.