Prof. Dr. Leon Neil Cooper > Research Profile
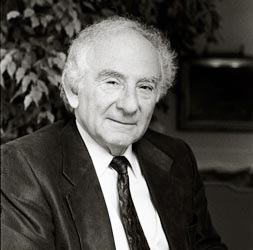
by Roberto Lalli
Leon Neil Cooper
The Nobel Prize in Physics 1972 together with John Bardeen and Robert Schrieffer "for their jointly developed theory of superconductivity, usually called the BCS-theory".
A Beginning in Nuclear Physics
Leon N. Cooper was born on February 28, 1930, in New York City. Since an early age, Cooper was interested in scientific topics, and as a teenager he set up a small laboratory for photography, chemistry and electrical stuff at a foster home in Mount Vernon. His interests in science, and especially in biology, were later encouraged when he frequented the famous Bronx High School of Science, from which he graduated in 1947. There, Cooper worked hard to obtain the necessary grades that would allow him to enrol at Columbia University. In February 1947, only three graduates from the Bronx High School of Science were admitted in that college, and Cooper was one of them thanks to the fact that he had won with an experiment on bacteria the Westinghouse Science Talent Search (from 1999 Siemens Competition)—a competition for high schools seniors established in 1939 to encourage talented students to pursue careers in science, mathematics, engineering, and medicine. Although primarily interested in biology, Cooper decided to major in physics, because he was convinced that physics was the science that would allow him to gain an understanding of the deep ideas concerning the building blocks of natural phenomena, especially relativity and quantum physics. After having majored in physics at Columbia University, Cooper began pursuing his PhD in theoretical physics at the same institution in 1951. He worked on the theoretical understanding of the newly discovered mu-mesonic atoms—atoms in which one or more electrons had been replaced by muons. By 1952, it had been already experimentally demonstrated through cosmic-ray observations that mu-mesonic (or muonic) atoms could be manufactured by slowing to rest muons in condensed matter. Cooper was interested in providing the theoretical framework of the experimental research on these kinds of atoms that was being pursued by Leo J. Rainwater and the PhD student Val Fitch at Columbia. Cooper made his research under the supervision of the American theoretical physicist Robert Serber—who had earned his PhD under J. Robert Oppenheimer and had made major contributions to the construction of the first nuclear weapons at the Los Alamos Laboratory. While he communicated rarely with Serber, Cooper could closely cooperate with the postdoc Ernie Henley who was working on the same topic. The cooperation was very fruitful and they completed their analysis in a short time with the publication of the paper “Mu-Mesonic Atoms and the Electromagnetic Radius of the Nucleus” in Physical Reviews. Since Cooper’s work on his theoretical program was solved so quickly he was asked to make other research before earning his PhD, including the theoretical study of multiple-scattering distribution along with Rainwater.
From Nuclear Physics to Superconductivity
After having earned his PhD in 1954, Cooper went to the Institute for Advanced Study in Princeton, which was becoming the mecca for recent PhDs in theoretical physics. Under Oppenheimer’s directorship, the institute came to be regarded as one of the most prominent places to build a solid experience in the most recent tools of theoretical physics thanks to the cooperative environment made of several highly talented postdoctoral fellows. Thanks to the work Freeman Dyson, the renormalization techniques developed by J. Schwinger, R. Feynman and S.-I. Tomonaga had been disseminated to a group of younger researchers, who were now contributing to the development of quantum field theory. At the Institute for Advanced Study, Cooper developed a strong interest for field theories and worked on nonrenormalizable field theories in order to understand their properties. While he was deepening his knowledge of quantum field theory, Bardeen contacted C. N. Yang—who was then a permanent member of the Institute—to ask for suggestion about a young scholar versed in field theory that could join his group to work on superconductivity. Yang proposed Cooper who was in fact attracted by the prospect to apply quantum field theory to the solid state, although he did not have any previous knowledge of solid-state physics. In September 1955, Cooper moved to Urbana-Champaign and began working with Bardeen and the PhD candidate Robert Schrieffer to a microscopic theory of superconductivity based on the laws of quantum mechanics.
The Cooper Pairs and the BCS Theory of Superconductivity
After superconductivity had be serendipitously discovered in the laboratory of H. Kamerlingh Onnes in Leiden in 1911, it was soon understood as one of the more interesting and unexplainable properties of solid state. The development of quantum mechanics in the mid-1920s and its success in the explanation of several properties of solids gave good reason to believe that superconductivity could also be explained as a quantum phenomenon due to the interaction of many particles. Theoretical tools to treat such many-body systems were developed gradually and, to some specialists, they appeared sufficient to provide a sound mechanism for the phenomenon according to which the resistance of some metals and alloys go abruptly to zero once their temperature goes below a certain temperature–called critical temperature. While experimentalists were improving the knowledge of the properties of superconductors (especially the Meissner effect, according to which superconductors are perfect diamagnets), theorists were not able to make much progress towards a quantum mechanical understanding of these various properties.
On the phenomenological side, however, some important steps were already made in the mid-1930s, when Fritz and Heinz London put forward the first theory of superconductivity that explained the relationship between supercurrents and magnetic fields. Bardeen—then a PhD student at Princeton—was one of the first scientists to recognize the importance of the London theory and to understand that to provide a microscopic theory of superconductivity one had to start with this theory. After further developments and the empirical understanding that the critical temperature depends on the mass of the ions for isotopic materials, Bardeen decided to intensively attack the unsolved problem to provide a microscopic theory of superconductivity.
Bardeen was not trained in quantum field theory, but recognized that quantum field theoretical tools might be essential to understanding the phenomenon of superconductivity as arising from the interconnections between an electron quantum gas and lattice vibrations. Just in the middle of this programme, Cooper was called to provide the necessary experience on quantum field theory. As soon as he arrived at the University of Illinois he offered some informal seminars on quantum field theoretical techniques; especially, Feynman diagrams corresponding to virtual excitations of the Fermi gas. Cooper, however, was worried that renormalization tools might not be the right approach to theoretically describe a phase transition, as the passage between normal and superconducting states was.
While teaching renormalization techniques to his co-workers, Cooper educated himself about superconductivity. In doing that, Cooper had the intuition that it was necessary to attack the problem with more simple techniques than those offered by the quantum field theoretical approach. To solve the problem of finding the quantum mechanical properties of degenerate systems, Cooper decided to look for the simplest case and, only later, to generalize the result to many-body system. In agreement with Bardeen, Cooper investigated the system made by two electrons just outside the Fermi surface to understand which were the properties that would have led to the emergence of the Meissner effect. Starting from quantum mechanical considerations, in September 1956 Cooper demonstrated that in a degenerate Fermi gas—an ensemble of a large number of non-interacting fermions that occupy all the states up to the Fermi energy—if the net force between the two electrons is attractive (however small the attraction might be) they form a bound state whose energy lies just below the Fermi surface.
Although Cooper’s demonstration was valid for any kind of attraction between electrons, Herbert Fröhlich, and, independently, Bardeen & Pines had already proposed a mechanism for the emergence of the attraction between electrons that involved the interaction between electrons and phonons, the quanta of the collective excitations of the ions forming the lattice. The bound energy is very weak and only below certain temperatures—the critical temperature of the superconductors—does the attraction overcome the Coulomb repulsion between electrons. The set of all these considerations led Cooper to suggest in the paper “Bound Electron Pairs in a Degenerate Fermi Gas,” that the emergence of a collection of these bound states of electrons below the critical temperature might be the microscopic phenomenon responsible for superconductivity. The Cooper pairs were born.
The idea to look for bound electron pairs as the phenomenon underlying superconductivity was being investigated independently by J. M. Blatt, S. T. Butler, and M. R. Schafroth, working at the University of Sydney. The latter had focused on systems of spatially localized electron pairs that could be treated as bosons, in that their supposed size was less than the average distance between them. In 1955, they showed that these kinds of systems could explain the Meissner effect and the Bose-Einstein-like condensation at a critical temperature. The Cooper pairs, however, seemed fundamentally different from the boson-like electron pairs in that the distance between the electrons was much greater that the average particle spacing.
Cooper’s research endeavour was a momentous step towards the formulation of a microscopic theory of superconductivity. It was necessary, however, to find the ground state of the superconductors that incorporated the Cooper pairs. This was a very difficult task, and, for months, Cooper received several criticisms to his solution, which was considered too simple for a problem so complex as superconductivity was believed to be. Bardeen, Cooper and Schrieffer tried many techniques to go from a single pair to a many-electron theory, but they faced great difficulties given by the overlapping of the electron bound states where the spatial separation between the electrons forming a Cooper pair was relatively large compared to the interparticle spacing. Only at the end of January 1957, Robert Schrieffer was able to derive the wave function of the superconducting ground state employing the variational approach S.-I. Tomonaga had used for the description of pion-nucleon interactions. After Cooper and Bardeen recognized that Schrieffer’s wave function had many satisfying properties, the trio began working intensively to the derivation of all the empirically testable theoretical predictions of the theory. Bardeen coordinated the division of labour, asking Cooper to work on the determination of the Meissner effect and other electrodynamic properties. It required six months to work out all the empirical consequences of their theory. However, worried that someone else (especially Feynman) could have derived a microscopic theory of superconductivity with a different method, Bardeen decided to publish a letter in Physical Review to secure priority for their discovery. On February 18, 1957, Bardeen sent to Physical Review the letter “Microscopic Theory of Superconductivity,” which contained the wave function and the main ingredients of the new theory. One month later, Cooper announced the theory with two talks at the Annual Meeting of the American Physical Society. In July 1957, after Bardeen had been able to derive the second order phase transition from normal to superconducting states, a 30-page article containing the full Bardeen-Cooper-Schrieffer theory was eventually submitted for publication in Physical Review.
The paper demonstrated how their theory was able to explain several of the empirical properties of superconductors up to then discovered. From that moment onward, the BCS theory—as the Bardeen-Cooper-Schrieffer theory came to be known—became the major candidate for the microscopic description of superconductivity, although serious doubts were raised about its physical implications by some theoretical physicists. Bardeen, Cooper and Schrieffer worked several months to reply to the criticisms exposed by other theorists who had been working on superconductivity. One of the most serious criticisms was that the theory seemed to lack gauge invariance, which could have implied a non-conservation of the number of electrons. This problem was solved within a few years by means of the work of various theoretical physicists. These advances led to the concept of broken symmetry, which became later relevant also in particle physics, especially in the formulation of the so-called Higgs mechanism—put forward by Peter Higgs and other theoretical physicists in 1964. After Cooper left the University of Illinois in 1957, he continued to work on the details of the BCS theory of superconductivity, its theoretical implications and its relation to experimental evidence.
The better understanding of broken symmetry and further experimental confirmations of its theoretical predictions led to strengthen consensus on the BCS theory as the standard approach to superconductivity. Particularly persuasive was the experimental discovery that the helium isotope helium-3—which is a fermion—becomes superfluid when it is cooled down below a certain temperature. This discovery gained the 1996 Nobel Prize to David M. Lee, Douglas D. Osheroff and Robert C. Richardson, who made the discovery at the low-temperature laboratory of Cornell University in the early 1970s. The superfluid properties of helium-3 could be explained as a consequence of the BCS theory, and Cooper had published with his co-workers Andrew Sessler and Robert Mills one of the first papers suggesting that helium-3 could become a superfluid below a critical temperature as early as 1959. Shortly after this discovery, Cooper, along with Bardeen and Schrieffer, was awarded the 1972 Nobel Prize “for their jointly developed theory of superconductivity, usually called the BCS-theory.”
Research in Neurosciences
After Cooper had received the Nobel Prize, he rediscovered his early passion for biology. As Cooper recalls, the deep switch was motivated by the fact that his theoretical research on superconductivity following the formulation of the BCS theory had become more and more technical, and no longer gave him much pleasure. Convinced that a theoretical approach could bring a better understanding of how the brain works, Cooper decided to immerse himself in the pioneering attempts to apply theoretical physics tools and methodology to neurosciences. He began formulating models of neural networks in animals and humans, with the main aim to provide a theoretical understanding of memory and other brain functions. As the director of the Brown University Center for Neural Sciences (later recalled Institute for Brain and Neural Systems) Cooper headed several interdisciplinary research groups of PhD students and associates interested in cognitive sciences. Among his major achievements in this field, one finds the formulation of the BCM theory of synaptic plasticity, which he formulated with Elie Bienenstock and Paul Munro in 1982. Cooper has been advocating an approach to neuroscience that favours a close contact between theories and experiments. Following this approach, the BCM theory is one of the most accurate theoretical accounts of experiments measuring the selectivity of neurons in the visual cortex. The theory constituted an essential improvement on the previous attempts to explain the process of learning and memory storage on a cellular and molecular basis—which substantially improved previous methods to stabilize the process of learning as a neural network put forward by the Canadian psychologist Donald Hebb in 1949. Since the early 1970s, Cooper’s papers and books have shaped the field and trained a new generation of students. To this day, Cooper is considered one of the pioneers of theoretical neuroscience and one of the greatest authorities in providing a vision of the possible direction of the entire field.
Bibliography
Anderson J., & Rosenfeld E. (2000) Talking Nets: An Oral history of Neural Networks. MIT Press, Cambridge, MA.
Bardeen, J (1956) Theory of Superconductivity. Theoretical Part, Handbuch der Physik 15: 274-369.
Bardeen J. (1972) Nobel Lecture: Microscopic Quantum Interference Effects in the Theory of Superconductivity. Nobelprize.org. Nobel Media AB 2014. Retrieved 10 December 2014. http://www.nobelprize.org/nobel_prizes/physics/laureates/1972/bardeen-lecture.html
Blais B. S., & Cooper L. (2008) BCM Theory, Scholarpedia, 3(3):1570.Cooper L. N. (1972) Nobel Lecture: Microscopic Quantum Interference Effects in the Theory of Superconductivity. Nobelprize.org. Nobel Media AB 2014. Web. 21 Jan 2015. http://www.nobelprize.org/nobel_prizes/physics/laureates/1972/cooper-lecture.html
Cooper L. N., & Feldman, D. (eds.) (2011) BCS: 50 years. World Scientific, Singapore.
Hoddeson, L. (1981) The Discovery of the Point-Contact Transistor. Historical Studies in the Physical Sciences 12: 41–76.
Hoddeson, L., Braun, E., Teichmann, J., & Weart, S. (eds.) (1991) Out of the Crystal Maze: Chapters from the History of Solid-State Physics. Oxford University Press, New York.
Hoddeson, L., and Daitch, V. (2002) True Genius: The Life and Science of John Bardeen. Joseph Henry Press, Washington, DC.
Leon N. Cooper - Biographical. Nobelprize.org. Nobel Media AB 2014. Retrieved 21 Jan 2015. http://www.nobelprize.org/nobel_prizes/physics/laureates/1972/cooper-bio.html
Schrieffer R. (1972) Nobel Lecture: Macroscopic Quantum Phenomena from Pairing in Superconductors. Nobelprize.org. Nobel Media AB 2014. Retrieved 10 December 2014. http://www.nobelprize.org/nobel_prizes/physics/laureates/1972/schrieffer-lecture.html