Prof. Dr. James Watson Cronin > Research Profile
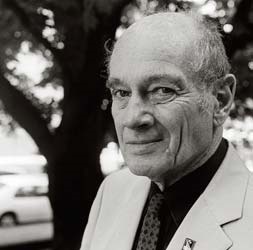
by Roberto Lalli
James Watson Cronin
Nobel Prize in Physics 1980 together with Val Fitch
"for the discovery of violations of fundamental symmetry principles in the decay of neutral K-mesons".
James Cronin was born on September 29, 1931, in Chicago, IL. His father, a classicist, was appointed professor of Greek and Latin at Southern Methodist University in Dallas when Cronin was in early childhood. Cronin’s family settled in Dallas, where Cronin was educated. The encouragement of a talented high school physics teacher was instrumental in strengthening Cronin’s inclination towards natural sciences. Initially interested in engineering, Cronin was convinced by his father to study physics at Southern Methodist University, from which he graduated in 1951.
Fascinated by the field, after graduation Cronin decided to pursue advanced study of physics, enrolling at the University of Chicago. In the early 1950s, the University of Chicago was very attractive for freshmen in physics. The Physics Department was at the forefront in research on nuclear and particle physics, and Cronin had the opportunity to attend lectures of many outstanding physicists of different generations, including E. Fermi, E. Teller, M. Gell-Mann, and M. Goeppert-Mayer. Cronin did his dissertation in experimental nuclear physics under the supervision of Samuel K. Allison, who had collaborated in the Manhattan Project. His doctoral research aimed at measuring the excitation functions and angular distributions of alpha particles in reactions between Boron and Beryllium. While working on his dissertation, Cronin developed a strong interest in particle physics. He was especially influenced by the views of Gell-Mann, who in that period was putting forward the hypothesis of the existence of a new quantum number—later called strangeness—to explain the properties of recently discovered particles (called K-mesons and hyperons). After Cronin had earned his PhD in 1955, he followed his interest in particle physics and joined the group of R. Cool and O. Piccioni at the Brookhaven National Laboratory (BNL). There, a powerful proton synchrotron—called Cosmotron—had recently begun operations accelerating proton beams up to 3 GeV. The BNL Cosmotron was the first machine to operate in the GeV range, enormously broadening the possibilities to explore the behaviour of particles at high energies. With its institution, particle accelerators rapidly displaced cosmic-ray observations as the preferred means to inquire into the realm of elementary particles: High energy physics (HEP) was born and Cronin entered this promising field.
Early discoveries with particle accelerators
In the early 1950s, particle physicists were dealing with several questions concerning the number and the properties of recently discovered elementary particles. Several of these particles had peculiar decay properties with respect to the previously known particles and were initially christened V-particles because of their two-particle decay mode. In order to classify the rapidly increasing number of ‘strange’ particles—as they soon came to be called—it was proposed to divide them in two groups: the K-mesons, whose mass was intermediate between the pion’s and the proton’s masses; and the hyperons, whose mass was greater than the neutron’s. When this general classification was proposed (1953), the exact number of K-mesons and hyperons was highly uncertain. Physicists were also puzzled by the fact that these particles, in particular the K-particles, were copiously produced during interactions, but had a surprisingly long lifetime. Gell-Mann’s 1953 strangeness scheme provided a possible explanation of this conundrum by hypothesising that the new quantum number was conserved in weak interactions, but violated in strong interactions.
Further experimental investigations on the properties of strange particles led physicists to identify an issue baptised the τ-θ puzzle. Two K-particles seemed to be identical in all their properties, but had different decay products (while τ decayed into three pi-mesons, θ decayed into two pi-mesons). This experimental finding could have been interpreted as two decay modes of the same particle. However, the different decay products entailed that τ and θ had opposed parity (P)—i.e., behaved differently under the transformation of mirror reflection. Since parity was believed to be an exact symmetry of nature, the τ-θ puzzle was regarded as a deep problem in the classification and understanding of strange particles.
In the spring of 1956, the Chinese-born theoretical physicists T.D. Lee and C.N. Yang found a way out of this dilemma by proposing that parity was violated in weak interactions. As the two physicists rightly pointed out, parity conservation had been well confirmed for electromagnetic and strong interactions, but there had been no unequivocal experimental evidence that this symmetry was also valid for weak interactions. Lee and Yang proposed several hypothetical tests for the conservation of parity in weak interactions, which were soon performed by several groups of experimenters employing the newly developed high-energy proton accelerators. At the beginning of 1957, a few experiments had already been completed. Their conclusion was that P-symmetry was in fact violated in weak interactions, which also implied the non-conservation of charge conjugation (C)—the operation of particle-antiparticle interchange.
The momentous discovery of the violation of what was considered one of the fundamental symmetries of nature soon led to several experimental investigations. In 1957, Cronin was involved in one of these tests. The American physicist D.A. Glaser had recently invented a new particle detector called bubble chamber. This device was an evolution of the cloud chamber—a detector that allowed a visualization of the trajectories of charged particles. Cronin collaborated with Glaser and other experimental physicists of the University of Michigan in a bubble chamber experiment investigating the violation of charge conjugation in the production of hyperons. In August 1957, the BNL-Michigan team completed the test confirming that C-symmetry was violated in the explored weak interaction. The enormous amount of striking experimental confirmations led to one of the quickest recognitions of the validity of a scientific achievement in the history of physics. In December 1957, Lee and Yang were jointly awarded the Nobel Prize in Physics “for their penetrating investigation of the so-called parity laws which has led to important discoveries regarding the elementary particles.” A few years later, in 1960, also D. Glaser gained the Physics Nobel Prize for the invention of the bubble chamber. Because of the possibility to visualize the actual trajectories of charged particles, the bubble chamber began to displace counter techniques as the preferred detector to investigate the properties of fundamental interactions at high energies.
Spark chambers enter the scene
The hegemonic role the bubble chamber enjoyed as the most appreciated particle detector for high energy events in American laboratories lasted only a few years. By the end of the 1950s, a new device came out of what the historian of science P. Galison labelled the “logic tradition.” The new instrument—christened spark chamber—allowed tracking the three-dimensional paths of charged particles by electronic means. The route that led to the invention and development of the spark chamber is very complex and included several researchers working independently in different sites in the same period. The summit of these research endeavours occurred in 1959 in Japan, where the physicist S. Fukui and his doctoral student S. Miyamoto succeeded in building an electronic device that reliably recorded three-dimensional trajectories of the incoming charged particles, developing the hodoscope chamber recently invented by A. Gozzini and M. Conversi.
In the United States, Cronin was among the first experimental physicists to recognize the enormous potentiali of the new detector. During his work at the BNL Cosmotron, Cronin had met V. Fitch, who invited him to join the Princeton University faculty in 1959. Left free to pursue his own research interests by Princeton Laboratory Director G. Reynolds, Cronin began constructing his own spark chamber, modifying the Fukui-Miyamoto design and employing a spark-counter developed by J. W. Keuffel in his doctoral thesis during the 1950s. In 1959, Cronin’s new spark chamber soon proved to be a very promising instrument for HEP experiments performed with proton synchrotrons. Besides maintaining one of the most important advantages of bubble chambers—the visualizability of charged particles—spark chambers were much more easy and inexpensive to realize. While constructing bubble chambers required the cooperation of large teams of 30-40 scientists and technicians, less than ten scientists were sufficient to build advanced spark chambers. In a matter of months, the new device caught the interest of several American physicists. After a visit to Princeton, a Columbia-BNL team decided to employ spark chambers instead of the bubble chamber in their quest for a second type of neutrino. In 1962, by employing the first large spark chamber attached to the BNL Alternating Gradient Synchrotron (AGS), this research led to the discovery of the muon neutrino. Spark chambers had suddenly and successfully entered the world of HEP research. In 1988, “for the neutrino beam method and the demonstration of the doublet structure of the leptons through the discovery of the muon neutrino,” Steinberger, Lederman, and Schwartz of the Columbia-BNL collaboration were awarded the Nobel Prize in Physics.
The discovery of CP violation
After the development of the spark chamber, Cronin himself employed the detector in research endeavours he pursued at the BNL Cosmotron, where he headed some teams studying of the properties of hyperon decays. In 1963, Cronin also completed an experiment aimed at the production and study of rho mesons—recently discovered short-lived hadronic particles whose preferred mode of decay was through the production of two pions.
Soon after, Fitch and Cronin began collaborating to explore in detail the properties of the neutral K-complex employing the spark-chamber tool. The neutral K-complex was a theoretical scheme put forward by M. Gell-Mann and A. Pais in 1953, which predicted the existence of two different neutral K-mesons with two different decay properties. The short-lived K-mesons (K1) decayed in two pi-mesons, while the long-lived K-mesons (K2) decayed in three pi-mesons. Upon the discovery of P-symmetry and C-symmetry violations in weak interactions, Landau proposed that a weaker symmetry was valid: the combined operation of P and C. The CP symmetry forbade that the K2 could decay in two pi-mesons. Until 1963, experiments on the properties of neutral K-mesons had confirmed that this was the case setting an upper limit of 1/300 for the branching ratio of the two-pion decay of the long-lived neutral K-mesons.
Fitch, Cronin and their co-workers—the French postdoc F. Turlay, and the graduate student J.H. Christenson—included the improvement of this upper limit in their research project, whose main focus was more general. After analysing their data in early 1964, the experimenters discovered that they had observed about 45 decays of the type K2=>2π in a sample of about 22,700 K2 decays. After further experimental confirmations and the rejection of possible alternative explanations, the experimenters reported that they had observed a tiny violation of CP symmetry (about 2x10-3) in the decay of the long-lived neutral K-mesons.
The discovery was first received with much scepticism because the belief in the CP symmetry was very widespread. However, in a few years a concomitance of various motivations—the convincing experimental evidence, further experimental confirmation by other groups, and the failure of theoretical alternatives to provide convincing arguments for the effect observed—led the community of physicists to accept that CP conservation was not an absolute symmetry of weak interactions and to explore the deep implications of this violation for the theory of fundamental interactions. After further theoretical evolution had led to the formulation of the Standard Model of electroweak and strong interactions by the early 1970s, Cronin and Fitch were awarded the 1980 Nobel Prize in Physics “for the discovery of violations of fundamental symmetry principles in the decay of neutral K-mesons.”
New technologies, new fields of study
After the momentous discovery of CP violation, Cronin continued to make active experimental research to elucidate the features of this violation. Cronin devoted several years to the observation of the decay of the long-lived neutral K-meson into two neutral pi-mesons. The study of this decay was particularly challenging because the CP-conserving decay of the long-lived K-mesons into three pi-mesons constituted a severe background. Moreover, the neutral pions rapidly decayed into two photons, whose range of energies prevented an accurate measurement of their direction and energy. In 1968, the Cronin group obtained an accurate result, which allowed a reliable evaluation of the parameters employed to measure the amount of CP-violating impurity in the two neutral states Ks—the new name of the short-lived K-meson after the discovery of CP violation—and Kl—the long-lived K-meson. In 1971, Cronin left Princeton University and accepted a position as professor of physics at the University of Chicago—his alma mater. The rationale underlying Cronin’s decision was that near Chicago a new particle laboratory was being constructed, which would shortly host the premier proton accelerator in the World. By 1973, the proton synchrotron of the National Accelerator Laboratory (in 1974 renamed Fermi National Accelerator Laboratory, better known as Fermilab) began operations accelerating proton beams up to 500 GeV. The same year, Cronin began performing experiments at the Fermilab aimed at measuring the branching ratio of the decay products of high-energy collisions. Cronin headed groups of experimenters for several years, collecting a large number of data. Particularly influential were the measurements of the cross sections for various charged decay products as a function of transverse momentum for several atomic targets and for different energies of the incoming protons. In 1975, these experiments led to the establishment of an empirical finding that was later labelled “Cronin effect.” The investigators observed that there was a significant enhancement in the production of high-transverse momentum hadrons for collisions pA with respect to collisions pp (scaled by the number of binary collisions), where A is the number of nucleons of the nuclear target and p stands for proton. This enhancement significantly increased with the value of A, signalling that bound nucleons behave in “a cooperative fashion” as the authors of the experiment suggested. The Cronin effect shortly produced a series of theoretical interpretations in the framework of quantum chromodynamics—the Standard Model theory of strong interactions that began gaining momentum around 1973. At the beginning of the 21th century, the effect aroused new interest because it was related to experimental observations on high-energy ion collisions carried out at the Relativistic Heavy Ion Collider RHIC (which began operations around the turn of the century).
After Cronin had received the Nobel Prize in December 1980, he carried out a series of experiments to determine the value of the parameters describing the CP-violating impurity of the neutral K-complex. While improving the precision of previous measurements, the data could not provide any decisive confirmation of the “Kobayashi-Maskawa mechanism.” In 1972, Kobayashi and Maskawa had discussed CP violation in the framework of the Glashow-Weinberg-Salam theory of electroweak interactions applied to hadron interactions. After being proved renormalizable in 1971 by G. ‘t Hooft and M. Veltman, the Glashow-Weinberg-Salam theory was immediately recognized as a promising model for the theoretical description of electroweak interactions. Elaborating previous works about the universality of weak interactions and the extension of the Glashow-Weinberg-Salam theory to hadrons—including the Cabibbo angle, the Glashow-Iliopoulos-Maiani mechanism, and a strong Japanese tradition of theoretical studies of particle interactions—Kobayashi and Maskawa demonstrated that CP non-conservation implied that at least three families of weak-interacting hadrons existed. When the two Japanese theorists published their proposal, the theory of quarks and gluons based on the 3-quark model was gaining momentum because of the discovery of asymptotic freedom by D. Gross, D. Politzer, and F. Wilczek. The theory of Kobayashi and Maskawa began gaining acceptance after the discovery of other particles, such as the fourth quark (charm) in November 1974 and the fifth lepton (tau lepton) around 1975. After the discovery of the fifth quark (bottom quark) in 1977, the three-family picture was increasingly employed in theoretical investigations, and received a strong confirmation when, in 1995, the discovery of the top quark completed the picture put forward by Kobayashi and Maskawa more than twenty years earlier. In 2010, Kobayashi and Maskawa were awarded the Nobel Prize in Physics “for the discovery of the origin of the broken symmetry which predicts the existence of at least three families of quarks in nature.”
In 1985, Cronin made his last experiment in a HEP laboratory. During a sabbatical year spent at CERN, Cronin was involved in the measurement of the lifetime of the neutral pion. After this experiment, Cronin turned his interest towards ultra-high energy cosmic-ray physics. Since 1939, rare events were registered in cosmic-ray observations that signalled the presence of extremely high-energy particles coming from outer space. Technological advancements made feasible the study of the origin of these particles by the late 1980s. Cronin has been involved in this kind of research since 1989, becoming one of the leading proponents of an international collaboration aimed at the study of these particles through the construction of very large particle detectors positioned at the surface of the Earth. The employed methodology is a modernized version of experiments performed in the 1930s by the French physicist Pierre V. Auger. Auger explored the energy and momentum of primary cosmic rays through the study of showers of billions of secondary particles produced by the collision of the primary ultra-high energy cosmic rays with the atmosphere. In 2003, a new giant detector array was completed in western Argentina— baptised Pierre Auger Observatory. Cronin currently serves as an emeritus spokesperson of the international collaboration aimed at understanding the origin of ultra-high energy cosmic rays through this new facility. According to Cronin, the understanding of the origin of these events will lead to new important discoveries in both astrophysics and fundamental physics.
Bibliography
Cronin, J. W. (1981) James Cronin - Biographical. Nobelprize.org. Nobel Media AB 2014. Accessed 30 Aug 2014. <http://www.nobelprize.org/nobel_prizes/physics/laureates/1980/cronin-bio.html>
Cronin, J. W. (1981) CP Symmetry Violation: The Search for Its Origin. Reviews of Modern Physics, 53(3), pp. 373-384.
Fitch, V. L. (1981) The Discovery of Charge-Conjugation Parity asymmetry. Reviews of Modern Physics, 53(3), pp. 367-372.
Fitch V. L. (1987) A Personal View of the Discovery of CP Violation. In Doncel, M. G. et al. (eds.) Symmetries in Physics. Universitat Autònoma de Barcelona, Barcelona, pp. 451-471.
Fitch V. L., & Rosner L. (1995) Elementary Particle Physics in the Second Half of the Twentieth Century. In Brown, L., Pippard, B., & Pais, A. (Eds.). Twentieth Century Physics (Vol. 2). AIP, New York, pp. 635-794.
Franklin, A. (1983) The Discovery and Acceptance of CP Violation. Historical Studies in the Physical Sciences, pp. 207-238.
Galison, P. (1997) Image and Logic: A Material Culture of Microphysics. University of Chicago Press, Chicago.
Hoddeson, L. Brown, L. M., Riordan, M. & Dresden, M. (1997) The Rise of the Standard Model: Particle Physics in the 1960s and the 1970s. Cambridge University Press, Cambridge.
Pais, A. (1986) Inward Bound of Matter and Forces in the Physical World. Clarendon Press, Oxford.