Prof. Dr. George de Hevesy > Research Profile
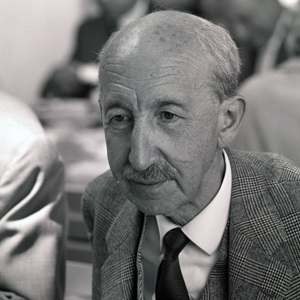
by Luisa Bonolis
George de Hevesy
Nobel Prize in Chemistry 1943
“for his work on the use of isotopes as tracers in the study of chemical processes”.
Isotopes as Radioactive Tracers in the Study of Chemical Processes
George de Hevesy was born in Budapest, Hungary on August 1, 1885, coming from a family of wealthy industrialists. He pursued his studies in physics and chemistry at the University of Budapest, and continued his education at the Technical University of Berlin earning his doctorate in 1908 from the University of Freiburg with a thesis in physical chemistry. He became an assistant at the Eidgenössische Technische Hochschule in Zürich, and later moved to the Technische Hochschule in Karlsruhe, to work under Fritz Haber, who was carrying out his epochal work on ammonia synthesis. In 1911 Hevesy joined Ernest Rutherford at Manchester in order to acquaint himself with radioactivity measurements.
At the time of Hevesy's arrival, Rutherford had just proposed his nuclear model of the atom, and soon the young Niels Bohr arrived from Denmark as a post-doctoral student. They were the same age, and formed a close lifelong friendship, which proved to be of decisive importance for Hevesy’s future life. Hevesy was also impressed by Henry Moseley, who would soon begin his pioneering research, applying the method of X-ray spectroscopy to the study of the X-ray spectra of various chemical elements.
In 1911, the main questions discussed in the Rutherford group concerned the structure of the atoms and the source of radioactivity, and scientists struggled to identify the decay products of the radioactive elements and to understand the relationship between the emission of different types of radiation and the change in “identity” of these products, i.e. their position in the periodic table.
One day Hevesy met Rutherford in the basement of the institute where some hundreds of kilograms of radioactive lead were stored, a gift of the Austrian government. It contained large activities of the valuable Radium-D. Rutherford said to Hevesy: “My boy, if you are worth your salt, you try to separate Radium-D from all that lead.” Hevesy worked hard at this task, trying over many months every chemical separation he knew. He failed completely and came to the conclusion that it was impossible to accomplish a separation through any chemical means. We now know that Radium-D is the radioactive isotope Lead-210, a form of lead with the same atomic number ---and hence the same chemistry--- as any other lead but with a different atomic weight.
After over a year of exhaustive disheartening effort, Hevesy thought of reversing the problem, as he himself reported in his Nobel Lecture: “In order to make the best of this depressing situation, I decided to use Radium D as an indicator of lead, thus profiting from the inseparability of Radium D from lead.”
Hevesy's imagination thus converted adversity into advantage and the concept of radioindicator chemistry was born.
Late in 1912, Hevesy returned to Hungary, mainly to obtain his Habilitation, i.e., the right to establish himself as a teacher and to give lectures at the university. On his way to Budapest he stopped at the Vienna Radium Institute headed by Stefan Meyer, where Friedrich Paneth had independently made unsuccessful efforts to separate Radium-D from lead. Hevesy proposed to Paneth to “mark” the non-radiating lead by Radium-D and then to investigate the behavior of its stable atoms by detecting and tracing the adjoining radiating isotope. He had actually devised an application of the isotopy concept, while Soddy was still fully formulating his theory according to which isotopes are elements belonging to the same position in the periodic table determined by their nuclear charge, but physically distinguishable because of their distinct mass and half life.
During the spring of 1913, Hevesy went back and forth between Budapest and Vienna and carried out the first radioactive-tracer experiments with Paneth, also showing that the electrochemical properties of radium-D were identical with those of lead, providing a further evidence to the existence of the isotopes. The collaboration with Paneth resulted in several papers, the first in which radioindicator tracers played a unique role in chemical analysis, establishing that radioactivity measurements could become a means of studying chemical processes which could not be observed any other way. In the spring of 1913, he returned for some time to Manchester. Between spring and early summer, Bohr submitted to Rutherford the first and second part of his paper on the constitution of atoms. In combining the quantum postulates with Rutherford's nuclear model, Bohr's theory predicted the wavelengths emitted in the spectrum of atomic hydrogen. In the meantime Moseley, who was at the time one of the most promising co-workers in Rutherford's laboratory, had moved to Oxford. He had investigated the characteristic beams of X-rays emitted by anticathodes of different elements when bombarded by cathode rays. Upon examining these rays by diffracting them through a crystal, he found a simple relationship between the wavelengths of the the X-ray produced and the atomic numbers of the elements used as targets. In arranging the elements not according to their atomic weights, but according to their atomic numbers, Moseley's law explained some anomalies in the periodic table and provided a justification based on physics for the sorting of the chemical elements. Moreover, it gave the possibility to look for so far undiscovered elements and provided a firm experimental evidence in favor of Niels Bohr's theory of the hydrogen atom, which had in the meantime been published in the well known trilogy of 1913.
Hevesy considered Moseley's method to be so important that he wanted to learn it. He had in fact planned to return to England in order to work with Moseley, who had invited him to work on the X-ray spectrum of the last members of the rare-earth group. But the political situation in Europe and the Balkan States was very unstable. The war broke out on August 1, when Hevesy was on vacation in Hungary, so that he returned to Vienna, later joining the Austro-Hungarian Army. He was never again to see Moseley who was killed in 1915 in the battle of the Dardanelles, at the age of 27.
At the end of the war, owing to the turbulent postwar political situation in Hungary, it was clear that there was no future for Hevesy in his country for many years to come. During the summer of 1919, he visited Bohr who had already invited him while they were together in Manchester. They agreed that Hevesy should return to Copenhagen in the spring of 1920, when Bohr's new institute - which was under construction - was to be opened. From a scientific point of view this was a unique chance for Hevesy, even if at the moment Bohr did not have much to offer as regards a more permanent position or salary. By that time Bohr had become a professor and his work was receiving general recognition. He was widely respected as the leader of the quantum revolution in physics and was loved for his deep humanness. Under his influence, the Institute would rapidly become a renowned world center for theoretical atomic physics and an international reference point for the scientific community.
The discovery of Hafnium
Hevesy arrived in Copenhagen in March 1920. At that time, Bohr had set out to determine the electron configuration of every element in the periodic table, an enormous undertaking that drew on every possible source: optical and X-ray spectra, atomic theory, chemical properties and the arrangement of the periodic system itself. Bohr suggested that the electrons around the atomic nucleus are arranged in shells and that only those electrons which are located in the outermost shell determine the chemical properties of any element.
When Bohr put forward these ideas, not all the places in Mendeleev's periodic table were filled. One of the missing elements possessed the atomic number 72. Element 71, lutetium, belonged to the rare earths. Chemists had long supposed that element 72 also belonged to that group, but according to Bohr, the rare-earth group is terminated with the element 71, and with seventy-two electrons a new orbital should instead open up. Consequently, element 72 cannot be a member of the rare-earth group. It ought to be a transition metal, similar to zirconium -- with virtually identical chemistry. This conclusion was stated in a footnote of Bohr's famous paper connecting chemical properties of elements with the structure of their atoms, published in 1922.
A variety of ideas and circumstances converged at this point. After Moseley's untimely death, the technique of X-ray spectroscopy had been further developed, and Hevesy still wished to learn it. Hevesy had also become interested in geochemistry, especially in the problems of the abundance of different elements and of the age of the earth. The rare-earth elements and their placement within the periodic system were topics of great topicality in this context. In September 1922, Bohr invited the physicist Dirk Coster from Holland, who had built a new model of X-ray spectrograph. Thus, the coincidence of Bohr's interest in the periodic law with Coster's arrival, the arguments about the placement of the unknown element either among the rare earths or as a zirconium homologue, Hevesy's newly awakened interest in geochemistry - all these factors called for a new initiative that might give experimental evidence for or against Bohr's views.
It was clear that the proper materials in which one should look for element 72 were minerals rich in zirconium, and that the amount of element 72 present in the mineral must be very small. Hevesy succeeded in obtaining a preparation which contained so much of the sought-after element that the X-ray spectrum of the sample very clearly showed the characteristic lines which could be calculated on the basis of Bohr's assumptions. This was a triumph for Hevesy and Coster who had overcome great experimental difficulties in order to prove that the unknown element 72 did not belong to the group of rare earths, but was indeed so similar to zirconium that it had lain well hidden up to that time. While their experiments were in progress, Bohr had been awarded the Nobel Prize. He announced their finding towards the end of his talk and the news spread fast all over Europe. The discovery immediately became important, being considered by many scientists, especially chemists, to be a confirmation of Bohr's theory of the periodic system.
The element was named Hafnium, after the Latin name Hafniae for Copenhagen, in honor of the home town of Niels Bohr, where the discovery was made and of which Hevesy was particularly fond.
Radioanalytical Methods in Biochemistry and the Birth of Nuclear Medicine
In 1923 Hevesy also returned to his work with radioactive lead tracers, and for the first time he ventured into biology and published the first study on the use of the naturally radioactive Lead-212 as radioactive tracer to follow the absorption and translocation in the roots, stems and leaves of Vicia faba, also known as the borad bean. Because of the extreme sensitivity of physical radioassay methods, he was able to carry out these experiments with such minuscule concentrations of lead as to avoid the toxic properties of it. In 1924, in collaboration with the Danish dermatologist Svend Lomholt and the chemist Jens A. Christiansen, Hevesy reported the first application of radioactive tracers to animal physiology. They used 5.0-day Bismuth-210 to label and to follow the circulation of bismuth in the bodies of rabbits after intramuscular injections of antisyphilitic medicaments containing bismuth. This investigation, which marked the first use of the tracer method in medical research, was instigated by the fact that bismuth was then being used in the treatment of syphilis, although very little was known about its mode of action.
In 1926, Hevesy was offered and accepted a professorship of physical chemistry at the University of Freiburg, where he remained from 1927--1934 continuing his biological studies, financially supported by the Rockefeller Foundation. There, with E. Alexander, he observed that when the elements of higher atomic number are bombarded with high-energy X-rays, a characteristic secondary emission of different energy begins that can be used in the detection and determination of the element in question. In this way, the method of X-ray fluorescence analysis was discovered. Later, the method became widely used for elemental and chemical analysis, particularly in the investigation of metals, glass, ceramics and building materials, and for research in geochemistry, forensic science and archaeology.
When Hevesy's interest focussed on the age of the elements and of the earth, he felt that the known values of lead content in meteorites and rocks were incorrect and he wanted to improve their accuracy. For this reason he developed, in 1929, together with R. Hobbie, the isotope dilution method of analysis. They added to the solution of rock samples a known amount of the lead isotope radium-D in negligible amounts - but with known activity - and deposited the lead electrolytically as peroxide on platinum anodes. By measuring the amount of radioactive isotope and the amount of stable isotope present and determining their ratio, both the quantity and mass of the element in the original sample could be ascertained. The isotope dilution method found by Hevesy within geophysical investigations could be applied to the investigation of the cosmic abundance of the chemical elements, but it could be employed more generally in analytical chemistry, in situations in which precise quantitative analysis by conventional means is difficult. He devised a most interesting application of the method in 1932, when Harold Urey discovered deuterium, one of two stable isotopes of hydrogen. Hevesy well knew Urey, who had spent 1923 at the Bohr Institute. Urey very generously supplied Hevesy with a few liters of water containing 0.6 per cent heavy water. Hevesy used it first to study the interchange of the water molecules of the goldfish with those of the surrounding water and later, introducing the principle of isotope dilution, studied the water content of the human body and the mean life time of the water molecules in the body. But these interesting investigations were interrupted by new dramatic events affecting Hevesy's life and career.
Artificial Radioactive Isotopes and the Neutron Activation Analysis
The Nazis came to power in early 1933. The racial laws affected Hevesy, and at last, in July 1934, once again homeless and uprooted, he returned to Copenhagen, where he enjoyed the warm hospitality of the Bohr Institute, and was able to readjust to a complete reshaping of his existence together with his family.
The move proved to be very fortunate for his research. During the time Hevesy had been a professor of physical chemistry in Freiburg, nuclear physics had progressed at an enormous pace.
In early 1934, Frédéric Joliot and Irène Curie in Paris had in fact produced the first artificially radioactive elements by means of alpha-particle irradiation. A couple of months later, Enrico Fermi and his collaborators used a Radon-Beryllium neutron source in Rome in their discoveries of a large number of radionuclides of many elements. Among them was 14.3-day Phosphorus-32, whose lifetime was long enough to study the role of an element central to all animal physiology, and a means of following its intake, circulation, exchange, and excretion.
“Chance only favors the prepared mind,” wrote Louis Pasteur. Hevesy's previous pioneering experiences with applications in biomedicine of natural radionuclides (and also of one stable isotope) had prepared him fully and well to recognize the potential applicability of this convenient radioactive form of a vital element for extending his radioindicator method to studies of diverse natural metabolic processes. Within a year after Phosphorus-32 was discovered, he published with Ole Chievitz (chief of surgery at the Finsen Hospital in Copenhagen) the first application of artificial radioactive indicators in the life sciences. The authors made a rather sweeping statement which was not in agreement with the views widely held at that time: “The results strongly support the view that the formation of the bones is a dynamic process, continuously taking up phosphorus atoms which are partly or wholly lost again and are replaced by other phosphorus atoms…”. While Hevesy's earlier application of the indicator method was of interest mainly to analytical chemistry, this pioneer experiment was a signal to biologists. A number of discoveries followed from the use of this tracer, which form the foundation of our understanding of body chemistry.
In the meantime, Hevesy immediately drew upon Fermi's method of transmuting elements through neutron irradiation for analytic purposes. With his co-worker and assistant Hilde Levi, a Jewish refugee from Germany with a Ph.D. in physics, he developed the method of neutron activation analysis, which later became a most important procedure for testing materials. Chemists in many countries were still laboring with the complex problem of the separation and purification of the different elements within the group of rare earths using the classical methods of analytical chemistry. Hevesy suggested that - as a means of identification - one could make use of the characteristic decay period of each of these elements and of their relative intensities of activation after neutron bombardment. In this way, even a minute amount present as an impurity could be determined in any unknown mixture of rare earth elements. These pioneering experiments were published in 1936.
At that time it was becoming clear that the production of radioisotopes could be enhanced manyfold if bombardment was performed in a high voltage accelerator or, especially, in a cyclotron like the one that had been built a few years earlier by Ernest Lawrence in Berkeley. A detailed description of a project entitled “physico-biological studies” and an application for funds was prepared by Bohr, Hevesy, and August Krogh, the well-known Danish physiologist and enthusiastic supporter of Hevesy's plans, and submitted to the Rockefeller Foundation. A cyclotron was built and became operational at the end of the 1930s, one of the first of these machines in Europe.
However, on April 9, 1940, Germany occupied Denmark. Bohr and his family were in danger, both because he was half-Jewish and an atomic scientist, and especially because he was an ardent and influential opponent of the Nazi regime. He was strongly urged by his friends to give up his stern refusal to leave his country. He was brought to Sweden on 30 September 1943 and a few days later he was flown to England. Hevesy, who had an Hungarian passport, simply boarded a train in Copenhagen and in mid-October appeared in Stockholm, where he continued his work with radioactive tracers in biology, physiology and medicine at the Institute for Organic Chemistry.
In 1944 Hevesy was awarded the 1943 Nobel Prize for Chemistry “for his work on the use of isotopes as tracers in the study of chemical processes,” and in 1958 he received the Atoms for Peace Award for his peaceful use of radioactive isotopes.
Bibliography
George de Hevesy (1962). Adventures in radioisotope research. The collected papers of George Hevesy. New York: Pergamon Press. Available at: http://openlibrary.org/books/OL23269162M/Adventures_in_radioisotope_research
John. D. Cockcroft (1967). George de Hevesy. 1885-1966, Biographical Memoirs of Fellows of the Royal Society 13: 125-166
Ferenc Szabadvary (1981). Hevesy, György. Complete Dictionary of Scientific Biography Vol. 6. Detroit: Charles Scribner's Sons: 365--367
Levi, Hilde (1985), George de Hevesy: life and work: a biography, Bristol: A. Hilger
Siegfried Niese (2009). Georg von Hevesy: 1885 - 1966. Wissenschaftler ohne Grenzen, Münster/Westfalen: Principal-Verlag
Helge Kragh (1980). Anatomy of a Priority Conflict: The Case of Element 72, Centaurus 23(4): 275-301
Eric R. Scerri (1994). Prediction of the Nature of Hafnium from Chemistry, Bohr's Theory and Quantum Theory, Annals of Science 51: 137-150