Prof. Dr. Val L. Fitch > Research Profile
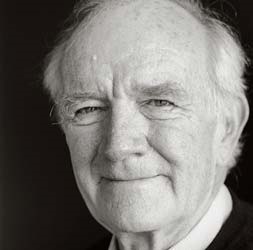
by Roberto Lalli
Val Logsdon Fitch
Nobel Prize in Physics 1980 together with James Cronin
"for the discovery of violations of fundamental symmetry principles in the decay of neutral K-mesons".
The first encounter with physics in World War II
Val Logsdon Fitch was born on March 10, 1923, on a cattle ranch in Cherry County, Nebraska, a few kilometres from the South Dakota border. Interested in the practical applications of science since his youth, Fitch actively entered the world of physics during World War II. In 1943, he was assigned to the Special Engineering Detachment at Los Alamos, the core research site of the Manhattan Project. At Los Alamos, more than five thousands of physicists, engineers, and technicians headed by first-rate scientists were assembled to work on the development of the first nuclear weapons. Under the direction of the English nuclear physicist Ernest W. Titterton, Fitch had his first direct experience of the problems related to experimental physics. He also had the opportunity to meet several leading figures of physics, including Bohr, Fermi, Chadwick, and Rabi. As Fitch himself stressed in his Nobel autobiographical note, at Los Alamos he learned the techniques of experimental physics and understood the relevance of electronics and electronic techniques in the development of experimental set ups. He also clearly realised that the central responsibility of experimental physicists was to invent new apparatus in order to measure new phenomena. His three years at Los Alamos convinced Firch to deepen his studies of physics and start a career in experimental physics.
Research on mu-mesonic atoms
After majoring in electrical engineering at McGill University in 1948, Fitch pursued his PhD studies at Columbia University under the direction of Leo James Rainwater—who would share with Aage N. Bohr and Ben R. Mottelson the 1975 Nobel Prize in Physics “for the discovery of the connection between collective motion and particle motion in atomic nuclei and the development of the theory of the structure of the atomic nucleus based on this connection.” The subject of Fitch’s doctoral research was the investigation of atoms in which one or more electrons had been replaced by muons. By 1952, it had been already experimentally demonstrated through cosmic-ray observations that mu-mesonic (or muonic) atoms could be manufactured by slowing to rest a muon in condensed matter. In his dissertation, Fitch focused on the exploration of the properties of such atoms by means of the detection of the gamma rays emitted by the muons when passing from one stationary state to another. Fitch designed and built a sodium iodide crystal scintillation spectrometer with tallium activation (NaI(Tl)), able to measure the energy of the gamma rays emitted by different muonic atoms, with atomic numbers ranging from 13 to 83.
As the theoretical physicist J. A. Wheeler had recently argued, the measurement of the transition energy 2p=>1s of the muon in a muonic atom could give valuable information about the diameter of the nucleus. Since muons are 200 times heavier than electrons, muonic orbits are much smaller than the corresponding electron orbits. By measuring the transition energy 2p=>1s of various muonic atoms created by means of the newly built Nevis cyclotron, Fitch and Rainwater provided a reliable measurement of nuclear diameters, under the assumption that nuclear density was constant inside a sphere of radius R. The conclusion of their experiments was that the diameter of the lead nucleus was about 1,2x10-13 cm. This value was about 15% smaller than that previously estimated with other nuclear-size-sensitive experiments. The report of the Fitch-Rainwater experiment was published in Physical Review in November 1953, and a few months later this work earned Fitch his PhD in physics.
Discrete symmetries and ‘strange’ particles
Upon the completion of his PhD, Fitch joined Princeton University, where he stayed till his retirement in 2005. His work at Princeton focused on exploring the properties of the recently discovered K-mesons (or kaons). In 1947, cosmic-ray observations revealed that there were more particles than previously believed. These new particles—initially called V-particles because of their ‘strange’ two-body decay properties—puzzled the physicists. There was no theoretical framework ready to explain their existence. Moreover, the experimental facts themselves were controversial. There was no agreement about the number of these particles or about their properties. Even their very existence was put into doubt for a few years after the V-particles were first detected in 1947.
In the 1953 Bagnères-de-Bigorre conference, it was decided to classify the newly discovered particles into two large families: The first group included the particles (called K-mesons) with mass between that of the pion and that of proton, while the second set comprised the particles (called hyperons) whose mass was greater than the neutron’s.
Once consensus was reached about their existence, the unexpected decay properties of the strange particles made them a central object of study. In 1953, the American theorist M. Gell-Mann proposed the existence of a new quantum number that was different from zero in K-mesons and hyperons and zero for the other particles then known (namely, the nucleons, the leptons, and the pion). Strangeness, as this new quantum number was later called, allowed for a classification of K-mesons and hyperons that worked remarkably well and predicted the existence of other, still undiscovered strange particles. The hypothesis that strangeness was conserved only in strong and electromagnetic interactions, but violated in weak interactions, could explain why the K-mesons were copiously produced but decayed weakly—one of the most troubling features of the ‘strange’ particles.
In 1953, a paper written by L. Leprince-Ringuet and B. Rossi summarizing the properties of the K-mesons prompted Fitch to think of experiments to elucidate the properties of these particles. The general picture that emerged from the Leprince-Ringuet-Rossi review paper was that there was considerable confusion about the decay properties of the kaons. The different observed decays led to the hypothesis that there were three different kinds of kaons. Leprince-Ringuet and Rossi concluded the article stressing the need for new experiments in order to establish whether different kinds of kaons really existed and to determine their properties with increasing precision.
Fitch’s early years at Princeton marked the passage from the “romantic age” of cosmic-ray observations on mountains to the work with the recently developed high-energy particle accelerators. After a discomforting year spent on Mount Evens (Colorado), Fitch began collaborating with the graduate student R. Motley on an experiment on the lifetime of K-mesons conducted at Brookhaven National Laboratory (BNL). They could employ the BNL proton synchrotron called Cosmotron, which by that time was operating in a regime of 3 GeV, becoming the first machine to reach the GeV energy range. In the summer of 1955, Fitch and Motley began performing the experiment with counter techniques, employing a Cerenkov counter.
Fitch decided to focus on the property of tau—a charged K-meson that decayed into three pions. When Fitch began measuring the lifetime of this particle, the particle tau was at the core of the most controversial interpretative problem about the K-mesons. Around 1953, Rossi had suggested that particles having the same lifetime and the same mass should be considered identical even if their decay products were different. The K-meson called θ, which decayed in two pions, had been measured to have the same mass and the same lifetime as the tau. Therefore, according to Rossi’s criterion the θ and the tau could be considered as two decay modes of the same entity. The problem was that the different decay products (2 pions against 3 pions) implied that the tau and the θ behaved differently with respect to one of the discrete symmetries of physics: parity. The parity transformation is the change of the sign of one spatial dimension, corresponding to the operation of mirror reflection—an operation that was denoted with the letter P in quantum mechanics. The belief that the laws of physics should be identical under spatial reflection—namely, that P should be an exact symmetry of nature for all fundamental interactions—was very strong. No evidence that this symmetry was violated was known in the mid-1950s.
In 1954, the spin-0 pion had been demonstrated to have odd intrinsic parity (P=-1)—i.e., the pion’s wavefunction was anti-symmetric under spatial reflection. From this property of the pion, it was possible to deduce in a straightforward way the parity properties of both tau and θ, which also had spin 0. It was rapidly understood that if parity was really a fundamental symmetry of all interactions, the parities of tau and θ were opposed: While the tau had the same parity as the pion (odd), the θ necessarily had to have opposed parity (even). As a consequence, tau and θ seemed to be identical in any respect but parity. As soon as the properties of the tau and θ were measured with increasing precision, physicists came to agree that they were facing a deep problem, which was named the tau-θ puzzle. With his precise measurements, with counter techniques, of the lifetime of the tau at BNL Cosmotron, Fitch contributed to the establishment of this puzzle: the lifetimes of tau and θ were measured to be virtually identical.
In the spring of 1956, the puzzle was solved in a way unforeseen by the great majority of physicists. The Chinese-born theoretical physicists Lee and Yang put forward the hypothesis that parity was not conserved in weak interactions—the interaction governing the decay of K-mesons into pions—and proposed a series of possible tests of the violation of parity. By the beginning of 1957, various groups of experimenters confirmed that parity was in fact violated in weak interactions. Moreover, Lee and Yang also deduced that the non-conservation of parity implied that charge conjugation symmetry C—namely, the invariance with respect to particle-antiparticle interchange—was also violated in weak interactions.
After a proposal by the Soviet theoretical physicist Lev D. Landau, in 1957 physicists came to agree that the combination of parity P and charge conjugation C was the deeper symmetry conserved in weak interactions—a property that was denoted CP symmetry.
Another discrete symmetry of nature (time reversal T) was also believed to be valid for all interactions. The combination of the three transformations, in any order, also formed a symmetry that was demonstrated to be strongly related to the foundations of the quantum theory of fields. The CPT theorem, stating that all physical laws are invariant under the combined operation of space reflection, charge conjugation and time reversal, implied that if CP symmetry was valid for any interaction also T should be an exact symmetry of nature. This was the general picture on which physicists had reached consensus by the end of 1957, following the groundbreaking discovery of parity violation in weak interactions, for which Lee and Yang were immediately awarded the Physics Nobel Prize in December 1957.
Neutral K-mesons, spark chambers and the discovery of CP symmetry violation
After his contribution to the establishment of the tau-θ puzzle, Fitch continued to work on experiments aimed at investigating the properties of K-mesons. In particular, he was guided by a theoretical scheme proposed by Gell-Mann and A. Pais to interpret the properties of the neutral K-mesons. According to the strangeness scheme elaborated by Gell-Mann, and independently by the Japanese physicists Nakano and Nishijima, there should be two different neutral K-mesons (one with S=1, the other with S=-1), corresponding to a particle-anti-particle pair. Fermi pointed out that there was no way to distinguish between the two different neutral K-mesons by means of laboratory experiments because they had the same decay products. Basing their reasoning on the non-conservation of S in weak interactions, in 1954 Gell-Mann and Pais proposed that the two neutral K-mesons mixed to produce two combinations of particle states, which had different decay products and, consequently, different lifetimes. Therefore, these states could well be considered the observable particles more than the original neutral K-mesons. These two particles were called respectively K1, whose principal decay mode was the production of two pions, and K2, for which the two-pion decay product was instead forbidden. Since the two-pion rate of decay was much greater than the rates for alternative decays, the lifetime of K2 was hypothesised to be much greater than the lifetime of K1. Consequently, K1 was called short-lived neutral K-meson, while K2 was christened long-lived K-meson. Only the former had already been detected by the mid-1950s.
The long-lived neutral K-meson was first observed in 1956, and soon Fitch began employing his skills to the construction of new counter detectors for the decay products of K2s. In 1957, he completed an experiment performed with Panofsky and other co-workers, which confirmed the existence of the long-lived neutral K-meson and provided a precise measurement of its total absorption cross section.
In 1961, at BNL a new powerful accelerator started operations. The Alternating Gradient Synchrotron (AGS) was planned to reach energies greater than 30 GeV, becoming the most powerful proton accelerator in the 1960s. Fitch began, along with other experimental groups, to employ the new accelerator to explore the properties of elementary particles at higher energies. With his graduate student D. Cassel and other co-workers, Fitch explored the form factor of the pion in the years 1962-63. While working at BNL, Fitch met James Cronin who was performing with his group an experiment devoted to the production of rho mesons—recently discovered hadronic particles whose preferred mode of decay was through the production of two pions. Cronin had developed a detection technique employing the recently developed spark chambers, which Fitch considered very suitable for the detection of the decay products of the neutral K-complex.
In the late 1950s, further properties of the neutral K-meson complex had been theoretically predicted and experimentally confirmed. The most convincing evidence concerning the validity of the neutral K-complex scheme was the regeneration of short-lived neutral K-mesons. Since the lifetime of the K2 (τ2) was calculated to be about 500 times larger than the lifetime of the K1 (τ1), after a certain time t (τ1<t< τ2) a pure neutral K-meson beam should be constituted almost exclusively of long-lived neutral K-mesons. However, if the beam hits a thin plate, the strangeness-conserving properties of strong interactions entail a considerable regeneration of the K1 state. In 1961, the regeneration of short-lived neutral K-mesons had been observed at the 6 GeV Bevatron at Berkeley. The amount of regenerated K1 detected corresponded fairly well with the quantity predicted on the basis of theoretical arguments. However, in 1963 a similar experiment performed at the BNL Cosmotron reported an anomalously large cross section of regenerated K1s from the scattering of K2 with liquid hydrogen.
This unexpected result stimulated Fitch and Cronin to join forces in order to test this anomaly at higher energies at AGS, where a neutral line (neutron beam) had recently become available. In April 1963, Fitch, Cronin, and the French postdoc R. Turlay sent a proposal to the AGS Scheduling Committee to explore the properties of neutral K-mesons. Apart from the main goal of the experiment concerning the anomalous K1 regeneration, the investigators included other goals, including a better estimate of the limit for the reaction K2=>2π. This experiment was considered as a test of the validity of CP symmetry, because if CP was an exact symmetry of nature, this reaction was forbidden. Previous measurements had set the upper limit at a branching ratio of 1/300. By May 1963, the experimenters received the official approval of their proposal: They had the opportunity to employ the AGS for about 200 hours in the summer of 1963 to perform the experiment.
Fitch, Cronin, Turlay, and the graduate student J. H. Christenson planned to employ 100 hours for the CP violation experiment and to detect around 7500 K2 decays, which would have set an upper limit of 1/7500 in case no 2π decay would have been observed. Although they did not expect to find any CP-violating decay, the investigators gave a certain importance to this part of the experiment in the proposed plan.
To detect the products of the neutral K-meson decays, the experimenters employed the newly developed spark chamber. In combination with Cerenkov counters and scintillation counters, the spark chamber allowed for a study of the trajectory of the detected charged particles, and, consequently, for the calculation of their momenta.
After about 100 hours of measurements, at the end of June 1963, the group stopped the observational part of the experiment and began analysing the data. The data analysis of the first part of the experiment showed no sign of the anomalous K1 regeneration, thereby confirming the neutral K-meson complex theoretical scheme. However, their study of the two-pion decay of K2 resulted in a momentous discovery.
In early 1964, the investigators reported that they had observed about 50 decays of the type K2=>2π in a sample of about 22,700 K2 decays. The experimenters checked the unexpected result with a hydrogen bubble chamber attached to the AGS in 1964, and confirmed the existence of K2=>2π reactions. After rejecting possible alternative explanations for their findings—e.g., that the two-pion event was an outcome of the regeneration of K1—the experimenters published their paper arguing that the K2=>2π reactions had been observed, and, then, that the CP symmetry was violated in weak interactions. Moreover, the authors were able to calculate that the branching ratio of this decay was about 2.3x10-3; the rate of violation of CP was extremely small.
Within two weeks from this announcement, an Illinois group of experimenters, also working at AGS, confirmed the violation of CP in K2 decays. However, several physicists were unconvinced that weak interactions could violate the CP symmetry. The small violation of CP implied, because of the CPT theorem, that weak interactions also violated the T symmetry. The idea that the laws of nature were not invariant under time reversal was very difficult to accept. A number of theoretical physicists put considerable efforts into the attempt to explain the findings of Fitch and his co-workers in a CP-conserving way during the period 1964-65.
In 1965, Fitch was involved in the test of one of these alternative hypotheses. Fitch and his co-workers showed that there was a constructive interference between K1 regenerated decays and CP-violating decays. This experiment showed that although K2 presented predominantly a CP-odd state, there was a detectable admixture of the CP-even state. Cronin later emphasised the importance of this experiment, which Fitch performed with R. F. Roth, J. Russ, and W. Vernon. The results of the experiment ruled out the possibility that the particles observed in the Fitch-Cronin-Turlay-Christenson experiment were not really pions or that the two-pion decay was accompanied by the emission of a third very light particle.
By 1966, the majority of physicists came to accept that CP was violated in weak interactions, the alternative hypotheses being ruled out by experiments or considered as implausible. Although a relatively small deviation, the discovery of the team headed by Fitch and Cronin opened new perspectives on the understanding of particle interactions, and the exact origin of this non-conservation is still puzzling theorists.
In the 1970s, the Standard Model of particle physics interpreted the small CP violation as an effect from a phase in the so-called Cabibbo-Kobayashi-Maskawa matrix of weak interactions, based on the existence of three generations of quarks. Kobayashi and Maskawa generalized the Cabibbo matrix of weak interactions to provide a model for the CP violation in 1973—when the high-energy physics community was still debating the validity of the 3-quark model.
In the 1970s, several predictions of the theoretical scheme that came to be called the Standard Model of particle physics were confirmed by high-energy physics experiments. In particular, evidence for the existence of the charm quark and the bottom quark, were reported in 1974 and 1977, respectively. The discovery of the bottom quark gave a strong impetus to the acceptance of the CKM matrix as a reliable description of weak interactions, although the sixth quark (the top quark) was still missing. Although still unclear from a theoretical perspective, the CP violation in weak interactions was considered as a fact of nature by the late 1960s. In 1980, after precise tests had confirmed some of the most striking predictions of the Glashow-Weinberg-Salam unified theory of electroweak interactions, Fitch and Cronin were awarded the Nobel Prize in Physics “for the discovery of violations of fundamental symmetry principles in the decay of neutral K-mesons.”
It took many years of experimental work and technological developments to test the existence of the sixth quark predicted by the CKM matrix, and only in the mid-1990s the physics community agreed that the top quark had been detected. This confirmation led to the Nobel Prize in Physics given in 2008 to Kobayashi and Maskawa “for the discovery of the origin of the broken symmetry which predicts the existence of at least three families of quarks in nature.”
Bibliography
Cronin, J. W. (1981) CP Symmetry Violation: The Search for Its Origin. Reviews of Modern Physics, 53(3), pp. 373-384.
Fitch, V. L. (1981) Val Fitch - Biographical. Nobelprize.org. Nobel Media AB 2014. Accessed 24 Jul 2014.
http://www.nobelprize.org/nobel_prizes/physics/laureates/1980/fitch-bio.html
Fitch, V. L. (1981) The Discovery of Charge-Conjugation Parity asymmetry. Reviews of Modern Physics, 53(3), pp. 367-372.
Fitch V. L. (1987) A Personal View of the Discovery of CP Violation. In Doncel, M. G. et al. (eds.) Symmetries in Physics. Universitat Autònoma de Barcelona, Barcelona, pp. 451-471.
Fitch V. L., & Rosner L. (1995) Elementary Particle Physics in the Second Half of the Twentieth Century. In Brown, L., Pippard, B., & Pais, A. (Eds.). Twentieth Century Physics (Vol. 2). AIP, New York, pp. 635-794.
Franklin, A. (1983) The Discovery and Acceptance of CP Violation. Historical Studies in the Physical Sciences, pp. 207-238.
Galison, P. (1997) Image and Logic: A Material Culture of Microphysics. University of Chicago Press, Chicago.
Hoddeson, L. Brown, L. M., Riordan, M. & Dresden, M. (1997) The Rise of the Standard Model: Particle Physics in the 1960s and the 1970s. Cambridge University Press, Cambridge.
Pais, A. (1986) Inward Bound of Matter and Forces in the Physical World. Clarendon Press, Oxford.