Prof. Dr. Riccardo Giacconi > Research Profile
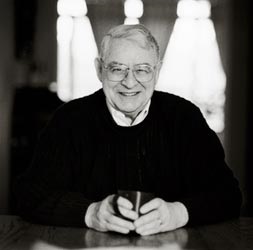
by Luisa Bonolis
Riccardo Giacconi
Nobel Prize in Physics 2002 together with Raymond Davis Jr. and Masatoshi Koshiba "for pioneering contributions to astrophysics, which have led to the discovery of cosmic X-ray sources".
A Training in Experimental Particle Physics
Riccardo Giacconi was born in Genoa, Italy, in 1931. His mother, a High School teacher of Mathematics and Physics, transmitted him her love for geometry. He chose to study physics at the University, even if he would have preferred becoming an architect, but he did not feel creative enough. From the first year, he became involved in cosmic ray research and worked with Antonino Mura and Carlo Succi, who studied muons, lambda particles, and proton interactions at the high mountain cosmic-ray laboratory Testa Grigia, in the Italian Alps. As a student, Giacconi was profoundly influenced by Giuseppe Occhialini, who had discovered with Patrick Blackett the phenomenon of pair production in the early 1930s. Occhialini had brought to Manchester the electronic coincidence counting of cosmic rays developed in Florence by Bruno Rossi. Blackett and Occhialini had married the Rossi circuit with the cloud chamber technique, of which Blackett was an acknowledged master. They had explicitly linked the pair formation to Dirac’s theory of the relativistic electron, placing it into a theoretical perspective. This achievement also confirmed the parallel discovery of the positron made by Carl Anderson and officially inaugurated the age of antimatter in the realm of physics. Repelled by the Fascist government, Occhialini left Italy for Brazil before World War II and was instrumental in starting cosmic ray studies there. After the war, he moved to Bristol and with his student Cesare Lattes participated with Cecil Powell in the discovery of the pion in 1947. Both Blackett and Powell were later awarded the Nobel Prize for Physics for their work in nuclear and particle physics. After a fruitful period in Bruxelles, Occhialini went back to Italy in the early 1950s and promoted in Milan activities in cosmic ray physics and later in space research. It was at that time that he exerted his influence on the young Giacconi with “his profound physical intuition, search for excellence and integrity in research, as well as his technical ability.” Occhialini encouraged Giacconi to apply for a Fulbright Scholarship and told him “go west young man,” a typical sentence from Occhialini's favourite cowboy movies.
Going West at the Beginning of the Space Era
In September 1956, after having done his thesis work in cloud chamber research on proton-produced interactions and having built a new very large multi-plate cloud chamber, Giacconi followed Occhialini's suggestion and joined Robert Thompson at the University of Indiana with a Fulbright Fellowship. He was fascinated by Thompson's style of working, and considered “his approach by building powerful instruments to achieve crucial results” a model. However, recalled Giacconi, “it became quickly apparent that Thompson's group was not the place for me. The search for the anti-lambda particle was unsuccessful and the new cloud chamber would take 10 years to build.” He then left Indiana and spent a year at Princeton with G. Reynold, during which he carried out an unsuccessful search for a new type of particle whose discovery had been claimed by Russian scientists. During this period, he learned a lot about scintillation counters and image intensifiers to be used for elementary particle research at the then-envisaged Princeton-Penn accelerator.
The work on scintillator chambers put him in contact with Herbert Bridge, a prominent figure of Bruno Rossi's Cosmic-Ray group at the Massachusetts Institute of Technology. At the beginning of the 1930s, Rossi had been the pioneer of cosmic ray research in Italy and one of the leading actors in the study of the nature and behaviour of the cosmic radiation. Up to the 1950s, Rossi had been one of the main investigators in the field of particle physics. However, by 1953, accelerator technology had developed to the point where energies comparable with those available in cosmic rays could be produced in the laboratory with known energies and directed precisely onto the chosen target. The interest in cosmic rays shifted to the question of their origin and of their propagation in astrophysical environments from their sources to the Earth. The existence of particles characterised by energies much higher than could be previously imagined revived the interest in mechanisms able to accelerate cosmic rays to such energies and further directed the attention of cosmic ray physicists towards outer space. The field of cosmic-ray research gradually transformed into two new fields, high-energy elementary-particle physics with accelerators, and cosmic-ray astrophysics.
As a young researcher, Giacconi lived through this transition period, which coincided with the advent of the space age, dramatically inaugurated with the launching on October 4, 1957, of Sputnik 1, the world’s first artificial satellite and the Soviet Union’s contribution to the International Geophysical Year. It provided the first measurements of the atmosphere density and tested the possibility of sending radio signals through the ionosphere. A full-scale political and cultural crisis resulted in the US from the launching of Sputnik 1, and the gap in the rush to space between the USA and the Soviet Union appeared even more dramatic when in November a second soviet satellite brought successfully into orbit the first living being, the dog Laika. On January 31, 1958, the first U.S. satellite, Explorer I, went into orbit, carrying a Geiger counter designed by James Van Allen’s group. This launch and the following mission discovered the existence of two layers of energetic charged particles held in place by the Earth's magnetic field. This first discovery inaugurated the era of science in space. By this time, it was very natural for Rossi's Cosmic-Ray Group at MIT to redirect a main part of their activities toward the new space-oriented program.
When his fellowship at CERN expired, Giacconi went to visit Occhialini at CERN: “He seemed to be in one of his emotional downturns seeing conspirators everywhere. CERN felt like an impersonal huge machine and offered me no prospects.” At that time Herbert Bridge was on leave from MIT at CERN. The big Proton Synchrotron would be ready to accelerate protons in November of that year, becoming for a brief period the world's highest-energy particle accelerator. It was an exciting atmosphere, but Occhialini's thoughts were wandering in a totally different dimension. With his wife Constance Dilworth, he soon joined Bruno Rossi at MIT where they spent the academic year 1959-1960. The contact with the new exciting research reality that was being pursued by Rossi's group eventually encouraged them to enter the field of space science. Occhialini eventually became one of the most powerful driving forces of European space missions. Within a few months, both Bridge and Giacconi, too, were destined to leave elementary particle physics, and to jump into the new dimension opened by the space age.
In the summer of 1958, Dwight Eisenhower had signed an act establishing the National Aeronautics and Space Administration (NASA), and at the same time the National Academy of Sciences created a Space Science Board to interest scientists in space research and to advise NASA and the other federal agencies the Academy expected to be engaged in space research. Bruno Rossi, as one of the most influential scientists in the United States, was among the fifteen members of the Board called together for their first meeting in New York on June 27, 1958. All the members were asked to form Committees promoting different research activities. Rossi was asked to form a Committee on special space projects. He immediately promoted the project of a space experiment for detecting cosmic gamma rays that members of his groups, George Clark and William Kraushaar, were planning as a natural extension of experiments with balloons. In April 1961, the satellite Explorer 11, carrying into orbit a gamma-ray detector, actually performed the first observation of high-energy cosmic gamma rays, with energies greater than 50 MeV.
At the same time, Rossi pointed out that no experiment had been devised to enquire about the hydromagnetic conditions of space around our planet and the Sun–Earth relation. Rossi felt thus motivated to initiate a study of interplanetary plasma with his own group at MIT, a project that led to the flight of Explorer X along the ‘‘tail’’ of the flapping magnetopause that took place in March 1961. The MIT plasma probe detected for the first time a steady, albeit variable, solar wind streaming past the Earth at supersonic speed and verified the existence of the previously hypothetical magnetospheric cavity, a region of space surrounding the Earth, which is shielded from the solar wind by the Earth’s magnetic field. This first flight prepared the stage for the complete vindication of Eugene Parker’s theory of the solar wind and inaugurated a new field of research, the study of the magnetospheres of other planets in the solar system and of the interplanetary plasma.
In July 1958, Rossi also suggested to the technical panel for the Earth Satellite Program that the study of X-rays of the high-energy group be given first priority in the early phase of satellite experiments. During the same period, Rossi's group was in fact planning balloon-experiments directed toward preliminary studies of X-rays from extra-terrestrial sources with the idea of undertaking a satellite experiment.
In 1959, the Sun was the only known celestial source of X-rays. It is a comparatively weak source, which can be detected easily from the Earth because of its proximity. For over ten years, solar X-rays had been extensively and successfully studied by scientists at the Naval Research Laboratory under the leadership of Herbert Friedman. Their experiment consisted of a collection of small Geiger counters aboard a captured German V-2 rocket, with which they were the first to detect X-rays from the very hot gas in the solar corona. However, the intensity of this radiation was found to be lower by a factor of 1 million than that measured at optical wavelengths. In the late 1950s, it was therefore widely believed that all other stars, much more distant than the Sun, should be so faint in X-rays that further observations at that energy range would be hopeless. On the other hand, results form high-energy cosmic ray experiments suggested there exist celestial objects, for example supernova remnants, which produce high-energy cosmic rays in processes that, in turn, may also produce X-rays and gamma rays. Evidence from ground-based radio astronomy showing that a number of galactic and extragalactic objects were more abundantly emitting at radio wavelength, than in visible light, led to the recognition that observable X-rays and even more energetic radiation should be generated through various physical processes. It seemed thus reasonable to expect the radio spectra to continue through the visible and ultraviolet, all the way to X-rays. At the same time, it was also that it would be technically challenging to detect X-rays emitted from extra-solar sources, whose fluxes might be so low that they could never be observed. Friedman himself had actually made unsuccessful attempts to observe extra-solar X-ray sources.
These topics were discussed during the meetings of the National Academy of Sciences and it was stressed that X-ray observations could yield important new astronomical information.
During a flight on the way home after a meeting of the Science Board, Rossi deeply reflected on the concrete possibility of detecting X-rays of extra-solar origin. He suddenly realised that soft X-rays, the lowest-energy radiation in the high-energy domain, could go through a certain thickness of air which can be compared to the mass absorption along galactic distances before being absorbed. He knew very little about astrophysics, but he was convinced that it was now possible, without any major technical improvement, to increase by a couple of orders of magnitude the sensitivity of the detectors used for solar observations without resorting to any fundamentally new technology. To be sure, the sensitivity of these detectors would still be a far cry from that deemed necessary for the detection of remote X-ray sources, recalled Rossi in his autobiography, “But no one had yet explored the sky with X-ray detectors as sensitive as those that I hoped could be developed, and this, for me, was a sufficient reason for undertaking this exploration; my long experience as a cosmic-ray physicist had taught me that when one enters an unexplored territory there is always a chance that he may find something unpredicted.”
As he had always done, Rossi discussed the idea with his colleagues of the MIT Cosmic-Ray Group. But they were heavily committed to the already mentioned important research projects: the study of the interplanetary plasma and the search for gamma rays from celestial sources, which had been initiated by William Kraushaar and George Clark. Still Rossi was not ready to forgo his plan, so he turned to his former student Martin Annis, the president of the newly founded company American Science & Engineering (AS&E), of which he was chairman of the board and chief science adviser. The company provided research services to several government areas, ranging from defence to medicine and to education. In particular, AS&E was working on projects related to the Atomic Energy Commission program of atomic tests, involving experiments for the measurements of X-rays and gamma rays from nuclear explosions, and had thus developed excellent capabilities for space missions and for developing detectors. Rossi, who was the chairman of the company, felt that AS&E should redirect its work toward peaceful pursuits. Moreover, he was hoping that an atomic test ban would be forthcoming and, in fact, he was working according to his capabilities toward this aim. In the absence of other outlets, the cessation of atomic tests would have created a difficult situation for the young company.
He was very excited when he talked with Martin Annis: “It just occurred to me that X-rays, specifically soft X-rays will penetrate the entire Universe without absorption. We have never looked through the window of the soft X-ray region of the spectrum.” Rossi told Annis that he wanted optics to focus soft X-rays to be developed, as well as large area detectors.
It was at that time that Giacconi, together with George Clark and Stan Olbert, the theoretician of Rossi's group, received an offer from AS&E through his acquaintance with Bridge, who was member of the scientific board. Bridge had been involved in the MIT plasma project, for which he had the main responsibility. He thought that Giacconi might be a good candidate for the company to hire and Annis's proposal was to utilise Giacconi's talents on any NASA supported program.
On his side, Giacconi felt he had not learned any useful skills while working in Milano, Indiana, and Princeton. On the other hand, he recalled, “It is fair to say that up to this point I had learned a lot of physics and techniques but I had not been very productive in research, nor had I found my real research interest.” He also desperately wanted some kind of permanent position. Moreover, AS&E offered an exciting prospect in the new area of research of space physics, and for these reasons he accepted the offer, hoping that this would be an occasion to learn a trade and provide the stability he needed for his family.
A Telescope for Cosmic X-rays
In September 1959, Giacconi joined the staff of American Science and Engineering and was given the task of working on the development of a program in space science with both defence and basic research applications. During a party, Giacconi met Rossi, who emphasised the importance of exploring the X-ray window on the Universe and suggested that Giacconi should concretely study the possibility of developing X-ray optics for this purpose. Rossi had a passion for optics, having just published an authoritative volume on the subject. In a few months of very intense work, Giacconi learned everything that was known about this not-yet-existing branch of astronomy and took up the challenge of developing an entirely new type of instrument. They were quite aware of the difficulties: considering the Sun to be a typical X-ray emitter, it became immediately obvious that the expected flux at Earth from celestial sources would be below the threshold of current detector systems by many orders of magnitude. ” This remained true even when considering the possible emission from hotter stars or supernovae. With Clark and Stan Olbert, Rossi examined the possibility that unusual X-ray objects, such as the Crab nebula or stars with high magnetic fields could be X-ray sources. And the results of this analysis were rather discouraging in the sense that, yes, there probably were X-ray sources, but very weak.
In studying X-ray properties and experimental techniques, Giacconi also read the Encyclopaedia of Physics edited by S. Flugge and went through early developments of the field. The problem of constructing a device to concentrate X-rays had been tackled for X-ray microscopy by Paul Kirkpatrick and by Hans Wolter. Wolter applied the well-known fact that X-rays can be reflected if they strike a smooth metal surface at a grazing angle. Because of their high-energy, X-ray photons that strike a mirror directly will penetrate into the mirror in much the same way that bullets aimed directly at a surface will bury themselves in it. Likewise, just as a bullets can ricochet off a surface when they hit it at a grazing angle, so too will X-rays ricochet off mirrors if they hit at very shallow angles, like a stone skipping across the surface of a pond. Wolter gave a theoretical demonstration of the possibility that X-ray images could be formed by a grazing incidence reflection over a parabolic mirror, followed by a second grazing incidence reflection over a hyperbolic mirror. X-rays do not reflect off mirrors the same way that visible light does. These properties mean that X-ray telescopes must be very different from optical telescopes. The mirrors have to be precisely shaped and aligned nearly parallel to incoming X-rays. Thus they look more like barrels than the familiar dish shape of optical telescopes
Giacconi studied the principles of X-ray optics and found all he needed to design the first X-ray telescope with an improvement in sensitivity of orders of magnitudes. When he first discussed this idea with Bruno Rossi, the latter suggested the key refinement of nesting several mirror surfaces one inside the other, thus increasing the effective collecting area and hence improving the detection rate. They immediately published a pioneering paper discussing the possibilities of constructing imaging X-ray telescopes. The first line of attack was thus the development of an X-ray telescope, even if early laboratory tests and a rocket experiment made clear that such a novel instrument would require a lengthy effort. NASA financed the proposal for constructing such an X-ray telescope: however, many years would have passed before a X-ray telescope would eventually fly.
The Birth of Extra-Solar X-ray Astronomy
In the meantime, they unsuccessfully tried to interest NASA in also funding exploratory flights of rockets with more conventional equipment, such as Geiger counters or proportional counters.
At that time there were good reasons to suppose that the Moon should be a source of X-rays. Two different processes were thought to be capable of producing X-rays on the lunar surface. The first was a scattering of solar X-rays. The second was the impact of high-energy electrons originating from the Sun. Therefore, Martin Annis, who was in charge of procuring funds as president of the AS&E, turned to the Lunar and Planetary Exploration Branch of the Air Force Cambridge Research Laboratories, who had funded previous work by AS&E in the classified domain. They looked favourably on a project aimed at detecting an X-ray emission by the Moon and agreed to finance the experiment in the hope that it would provide some information on the chemical composition of the lunar surface. They were given support to place a small Geiger counter on a flight.
The detectors used by Friedman's group were Geiger counters outfitted with thin mica windows of about one square centimetre in area that allowed X-rays to enter their sensitive volume. Already in June 1960, the group at AS&E, in collaboration with MIT, had launched a similar instrument from the Air Force Englin base, in Florida. The launch had failed because of a malfunction of the Nike-Asp rocket engine. The payload consisted of Geiger counters, shielded with an anti-coincidence system of scintillators, designed to substantially reduce the background, induced mainly by cosmic rays. In January 1961, they received a new contract to fly four Aerobee 150 rockets for the experiment to search for X-ray stars as well as lunar X rays. The larger rocket permitted the design of a much more sensitive instrument.
The response to X-rays depends on the area of the window. The most obvious method to increase the sensitivity of a counter was to increase the size of its X-ray transparent window. However, since it is not possible to increase this area substantially without increasing the dimensions of the counter, thereby increasing its sensitivity to cosmic rays, it is clear that no substantial increase in the effective X-ray sensitivity of a counter can be achieved by an increase of its window area. As Rossi had suggested, the most effective method was to selectively concentrate over a very small X-ray detector the X-rays incident over a large area of collection. The problem of increasing the effective area was solved by Frank Paolini, who had joined the group in 1960. He had the idea of realising a pancake structure for the counters with several mica windows to allow larger transparency to X-rays. Herbert Gursky was responsible for the detectors, three Geiger counters, each with an area of 10 cm2. Thin mica windows were used, which permitted detection of X rays between 2 and 8 angstrom. An important feature of the experiment was the use of a large field of view, which increased the probability of both observing a source anywhere in the sky and receiving a sufficient number of X-ray photons to make detection statistically significant. Rather than the 3° field used by Friedman for his extreme ultraviolet and X-ray searches, they used a field of view of 120°. As recalled by Giacconi, “The probability that a spinning rocket with a field of view of 3° passes over a source is 1%. With 120° it is 60%. Furthermore, a detector with such a small field dwells on a source (during a scan) for a correspondingly small time and thus collects a small number of photons, insufficient to distinguish a source from background noise. In effect, the payload we designed and flew was 100 times more sensitive than any flown until then.”
George Clark remarked that all this was done in “the typical Rossi exploratory style, that always characterised his work that he has had any relation to, and that is, in the first place, the imagination to go after something interesting, and then, to do it with a broad enough net so you have a fair chance to catch it.”
Lunar fluorescence from solar X-rays appeared as possibly the strongest source to be expected. However, while their experiment was to be officially aimed at the Moon, they hoped that it would also represent a first tentative step in the search for other much more remote X-ray sources. The new payload was launched onboard an Airforce Aerobee rocket just before midnight on June 18, 1962, from the White Sands base in New Mexico. The rocket was spinning with a period of 0.5 sec with the rotation axis oriented to Zenith. Only two detectors worked properly. The results were surprising but not totally unexpected: no X-rays were detected from the Moon. However an intense source of X-rays, by far more intense than any predicted celestial source, was observed in the Scorpius region together with an unexpected diffuse X-ray background.
The plot showing the azimuthal distribution of the count rates of the Geiger countera, published in Physical Review Letters on December 1962, signed by Giacconi, Gursky, Paolini and Rossi, marks the birth of X-ray astronomy. The truly extraordinary aspect of the discovery was not that an X-ray star had been found but its extraordinary properties. Here was an object that emitted a thousand times more X rays than the sun at all wavelengths and a thousand times more energy in X rays than in visible light! Clearly, they were dealing with a new class of stellar objects in which physical processes not known in the laboratory were taking place. The physical process by which the X-rays were emitted on Sco X-1 had to be different from any process for x-ray generation they knew in the laboratory. The background was also very interesting, because it might carry cosmological information.
Immediately after the seminal discovery of the mysterious X-ray source, Friedman’s group launched, in April 1963, an Aerobee rocket carrying a proportional counter 7 times more sensitive that the one of Giacconi and collaborators. The rocket scanned the sky, even if the galactic centre remained under the horizon, and observed two X-ray sources: one in the Scorpius region, Sco X-1, and a second bright source in the direction of the Crab Nebula. The existence of an isotropic X-ray background was also confirmed. This experiment cleared any residual doubt on the existence of an X-ray Universe. The cover of the November 1st, 1963, issue of TIME announced the birth of X-ray astronomy to the world: X Rays in the Unknown: “That object is spewing out more X rays than had been calculated to come from all the rest of the billions of stars in the galaxy put together...”
The AS&E group concentrated on the detailed study of individual X-ray sources. Important innovations were made within the first few years of the discovery flight. One was to switch to proportional counters from Geiger counters. Proportional counters allowed a crude measurement of the energy of detected photons and allowed for efficient background rejection techniques. A second innovation, the modulation collimator, allowing multiple transmission directions, was instrumental in the 1966 flight during which the X-ray source Sco X-1 was localised with arc minute precision, eventually leading to its optical identification and to the emergence of the recognition that binary systems with a collapsed companion star could be very luminous in X-rays.
Early Developments of X-ray Astronomy
On September 25, 1963, Giacconi with Gursky and collaborators submitted to NASA a proposal for “An Experimental Program of Extra Solar X-ray Astronomy,” a long term plan for the development of X-ray astronomy from rocket experiments to a dedicated satellite to imaging X-ray telescopes on the Orbiting Astronomical Observatory and finally to a 1.2 metre diameter X-ray telescope, which could image and detect the background with an angular resolution of 1 arc min. This strategic plan provided Giacconi's own view of how the field should develop, at a time when very few people had a clear vision of what X-ray astronomy could become. There was in fact still a great deal of resistance at NASA Headquarters and also in the scientific community to the development of telescopes for stellar astronomy, also because many thought that X-ray sources were likely to be extended and therefore there was no point in high-resolution studies. This youthful dream was not fully realised until the launch of the Chandra X-Ray Observatory in 1999, which, not by chance, had a 1.2 metre diameter mirror. But while the difficult technological development that made X-ray telescopes possible was being carried out, the most fundamental advances in X-ray astronomy were made with relatively crude detectors mounted on orbiting satellites.
The findings of the 1962 rocket flight stimulated an enormous amount of interest in the astronomical community both from the observational and the theoretical side. Efforts were aimed at analysing the possible sources of cosmic X rays and the production mechanisms that might operate in galactic and extragalactic space. As in the case of radio astronomy, it was soon clear that the X-ray Universe had very peculiar, unexpected and new features. Giacconi well emphasized how “One of the most interesting consequences of the early finding was that, as pointed out by Fred Hoyle and Burbidge, the background intensity observed in the X rays was so greatly below that expected for the hot universe model of the steady state universe as to make it untenable. This was the first real input of X-ray astronomy to cosmological questions.”
A number of rocket flights were carried out from 1963 to 1970 in order to discover additional sources and to answer the fundamental question of the class of objects corresponding to X-ray sources such as Sco X-1. All these experiments involved making optimal use of the few minutes of observation that were possible during each rocket flight. By 1967, more than 30 X-ray sources were known, including a number of supernovae, the quasar 3C273 and the radio galaxy M87. In about ten years, the number of publications on X-ray astronomy was more than one thousand.
The rocket experiments had shown that the spectra of compact X-ray sources are quite hard, extending much beyond 10 keV. This opened up the possibility of using balloon-borne instruments, as the atmosphere becomes transparent for altitudes above 40 km at high X-ray energies (>20 keV). During the 1960s, instruments were carried aloft by balloons, complementing the rocket X-ray studies. Balloon flights offered the advantage of long-duration observations (up to 100 h) and the crystal scintillation counters used in these experiments allowed spectra to be taken up to about 500 keV. Balloon observations were pioneered by the MIT group in the 1960s. In 1965, a balloon mission prepared by George Clark detected hard X-rays from the Crab nebula. Successful launches were performed in Germany by Joachim Trümpfer, Rüdiger Staubert and Eckard Kendziorra, observing tens of X-ray sources, including several black hole binaries and bright Active Galactic Nuclei. At the end of the 1960s, more than 30 celestial sources had been discovered to emit in X-rays. Key achievements had been obtained, including the observation of the first extragalactic source, M 87, a supergiant elliptical galaxy, that now is known to host a giant black hole whose mass has recently been estimated to be about 6 billion solar masses, or about 1500 times as large as the supermassive black hole in the Milky Way Galaxy.
The 1960s were an incredibly exciting period in astronomy: together with the X-ray stars and the X-ray background, the 3 K radiation, the pulsars, and the quasars were discovered. New observational capabilities were on the horizon. Major advances in the field began in the 1970s with the use of satellites equipped with X-ray detectors. The first of these, Uhuru, was launched in 1970.
Mapping the X-ray Universe with Satellites
In the 1960s Giacconi's group continued the study of stellar sources with counter experiments; in this enterprise, they were joined by many groups in the world. All these pioneering observations provided a glimpse of the richness of the X-ray sky, but the picture was still confused. These problems were resolved with the launch, in December 1970, of the Uhuru X-ray observatory, which was the first satellite dedicated to X-ray astronomy and which initiated the successful series of Explorer satellites sponsored by NASA. It made it possible to monitor X-ray stars for prolonged periods of time, instead of the five minutes above 100 km for each rocket launch. All aspects of the Uhuru science instrumentation were designed at AS&E.
The detection system consisted of two proportional counters with beryllium windows 2.5 mm thick, sensitive in the 2-10 keV energy range, arranged ‘‘back-to-back’’, and allowing an effective area of 840 cm2 each. The two units operated in anti-coincidence, assuring an instrumental background rejection of about 90%. The NASA engineer Marjory Townsend of the NASA Goddard Space Flight Center was appointed project manager of the mission. She was the first woman ever in such a position. The satellite was designed to rotate slowly on its axis, sweeping a 5° band of the sky every 12 minutes, that shifted by 1° per day. Uhuru scanned many times over the same region, thereby greatly increasing its sensitivity to weak sources. In three months the entire sky could be studied systematically and many new sources could be localised with a precision of about 1 arc minute, often permitting the identification of the x-ray sources with a visual or radio counterpart. This in turn led to an evaluation of the distance, the intrinsic luminosity, and the physical characteristics of the celestial object from which the X-rays emanated.
The satellite was launched from the Italian S. Marco platform, off the shore of Kenya, on the Kenyan freedom day and was named Uhuru, which means freedom in Swahili.
The speed of rotation, as well as the spin axis orientation, could be controlled from the ground. Star sensors permitted them to locate the source of X-ray emission in the sky. They arranged with NASA to send them a portion of the data through a telephone line each day. It was essential to the success of the mission, which depended on their ability to change their observing program in response to actual discoveries. As soon as the first orbit (just one orbit!) was completed, Uhuru had already revealed the existence of 30 X-ray sources, including 7 new discoveries. But even more important from a certain point of view was the ability, which was provided by the control system, to slow down the satellite spin and spend a very long time on an individual source to study its temporal variations. The nature of the variability of the sources was one of the key objectives of the mission. It was this special ability that permitted the solution of the fundamental unresolved problem of X-ray astronomy until then, namely, the nature of the energy source capable of producing the large intrinsic luminosity of the stellar x-ray sources.
Two eclipsing X-ray pulsars with Doppler-shifting period, Her X-1 and Cen X-3, demonstrated the existence of a class of binary X-ray sources consisting of a normal star and a spinning, magnetised neutron star. This second manifestation of neutron stars, closely following the identification of radio pulsars, revealed a whole new domain of binary system evolution. Furthermore, the source of energy for the system was immediately identified as accretion from the binary companion onto a neutron star. Accretion of matter in such a system is a very powerful energy source. Within a few months the extended observations had shown that variability was a common phenomenon among X-ray sources. This led to the historic discovery that many of the most luminous galactic sources were in binary star systems. It is with Uhuru that binary X-ray sources containing neutron stars and black holes were in fact discovered. It was found that the energy for the abundant X-ray emission from these sources was provided by the infall of gas from the companion onto the collapsed object.
A second Uhuru discovery, diffuse X-ray emission from several clusters of galaxies, suggested the presence of a dilute hot gas interspersed among the galaxies, an interpretation that led to important evolutionary studies. The detection of a rapid aperiodic temporal variability of the intensity of Cyg X-1 led to rocket and ground-based observations, which identified the optical counterpart, and to a determination of the mass of the accreting compact object. This mass turned out to be larger than the limiting mass of a neutron star thus suggesting that the source was a binary system containing a stellar black hole. These discoveries and numerous other pioneering studies by Uhuru brought X-ray astronomy solidly into the mainstream of astronomy.
When the mission finished in March 1973, an entire Universe had been unveiled. The science results of the mission were outstanding. Supernova Remnants and X-ray binaries hosting compact objects were established as intense sources of X-rays. Extragalactic sources included galaxies and clusters of galaxies, Seyfert galaxies and Quasars. The Uhuru catalogue published by Giacconi and collaborators resulted in 339 sources. The first half of the 70s was an era of fast growth for X-ray astronomy. The amount and quality of information produced by the Uhuru data created a quantitative and qualitative change, which brought X-ray observations into astronomy as a distinct discipline. Thanks to the discoveries of Uhuru, new theoretical models were developed to explain the physical mechanisms at the base of X-ray emission. X-ray observations were emerging so rapidly as a powerful tool for astronomy because of the prevalence of high-energy phenomena in the universe and the important and at times determining role that they play in its dynamics and evolution.
The Einstein Observatory
During the same period, Giacconi's team concentrated on the use of X-ray telescopes in solar X-ray astronomy, where there was an understood need to improve the angular resolution. The development of focussing optics that could produce proper images of X-ray emission stemmed from the key ideas expressed in the first pioneering article by Giacconi and Rossi. This was not only interesting scientifically, but allowed them to continue developing better and better telescopes which eventually could be used for stellar astronomy.
The group at AS&E now included Leon Van Speybroek and Giuseppe Vaiana, the first an outstanding optical designer and builder, the second a brilliant solar and later stellar astrophysicist. They designed a solar X-ray telescope with thick beryllium walls. The group provided a solar experiment flown on board of Skylab, the first manned US space station. During that period the required technology for an X-ray telescope were developed and first applied to studies of the sun, culminating in the many thousands of highly resolved pictures of the solar corona which the astronauts brought back from Skylab.
In the meantime, a consortium of institutions including AS&E, Columbia University, Goddard Space Flight Center, Massachusetts Institute of Technology and the Marshall Space Flight Center proposed to NASA an observatory called the “Large Orbiting X-ray Telescope” consisting of two telescopes of different type. The program was approved in September 1970, but was cancelled by NASA after three years of work, because of funding problems related to the Viking planetary program. NASA offered to fly only one telescope, of 0.6 rather than 1.2 m diameter, on a smaller spacecraft. A reduced version of the original project became what was later known as Einstein, the first orbiting observatory to carry a high resolution X-ray telescope. The grazing incidence telescope consisted of four nested paraboloids of revolution followed by four nested hyperboloids. The nearly cylindrical surfaces were nested inside one another to increase the effective collecting area, which in the end corresponded to slightly more than the area of the great Hale Observatory-Mount Palomar 200-inch optical reflector. The mirrors were made of fused quartz. A very thin nickel coating provided the desired index of refraction for focusing X-rays. Two reflections for each X-ray (one by a paraboloid and one by the following hyperboloid) allowed to achieve a reasonable resolution over the 1° field of view of the telescope. Two types of complementary imaging detectors were developed to be employed at the focus of the telescope. A new type of detector, the High Resolution Imager, was developed by Steve Murray of Giacconi's team, then at the Harvard-Smithsonian Center for Astrophysics, at Harvard. The position and time of arrival of each photon was measured and transmitted to the ground by telemetry. The coordinates of the detector were translated into sky positions by an on-board self-calibrating system. The Einstein observatory contained a number of imaging cameras and spectrometers on a rotary table that could be placed in the focus on command. A special test facility was constructed and used to test the fully integrated spacecraft with a quasi-parallel beam produced by a point-like source at a distance of about 300 m.
After performing a series of tests, Einstein was launched on November 13, 1978, and the results exceeded all expectations. The rich phenomenology of the high-energy sky revealed by earlier missions had only little overlap with the interests of the mainstream of traditional astronomy, since only exceptionally luminous galactic sources and only the nearest and most powerful extragalactic sources could be observed.
The situation changed radically with the launch of the Einstein Observatory, and with the introduction of focusing high-resolution optics to X-ray astronomy. This innovation resulted in a qualitative change in the scope of the discipline. At one stroke, X-ray observations were extended to all known classes of stars in our galaxy and to the most distant discrete objects known in the universe.
In producing high-resolution images and accurate locations for thousands of cosmic X-ray sources, the Einstein Observatory brought X-ray astronomy close to optical and radio astronomies as a major branch of observational astrophysics. Its large grazing incidence telescope superseded the sensitivity of earlier satellite instruments to a level where X-ray emission could be measured, not just from unusually powerful objects - such as X-ray binaries, Active Galactic Nuclei, clusters of galaxies and young supernova remnants - but from a wide range of “normal” stars and galaxies. It also made possible a new way to study the mysterious dark matter that surrounds many galaxies. As a matter of fact, it was realised that X-rays play a key role in the understanding of the formation and evolution of celestial objects.
Stellar X-ray astronomy essentially began with the Einstein Observatory. This forced Giacconi's team to make a significant effort to make the data available to astronomers in all disciplines in an immediately usable form quickly enough to allow for follow-up observations. From the start, they had also planned to make observing time available to guest investigators, so that some 2,000 out of 5,000 total observations were carried out on behalf of 150 guest observers around the world. Einstein thus became a truly international observatory and the program established a methodology that has been almost universally adopted since then in observatories both in space and on the ground, like Hubble, the ESO Very Large Telescope array (VLT) and Chandra. Some 1,000 papers appeared in refereed journals in the first 10 years after the Einstein launch.
Groups from many nations joined Giacconi's team in the use of focusing optics after the launch of Einstein in 1978. All the major X-ray missions conducted after 1990 used X-ray telescopes, particularly the satellite Rosat, by the Max Planck Institute group led by Joachim Trümper. Rosat had a larger mirror than Einstein (0.8 rather than 0.6 m), a wider field of view, and about the same angular resolution. Launched in 1990, Rosat proved invaluable for filling in the gap between the demise of Einstein in 1980 and the launch of Chandra and XMM-Newton in 1999. After nine years in space, an all-sky catalogue of 150 000 objects was compiled, many of these sources providing important targets for the Chandra and XMM-Newton Observatories.
The revolution in non optical-astronomy, in radio and infrared astronomy at long wavelengths and in gamma ray astronomy at short wavelengths was revealing that much of the universe lies beyond optical perception. The X rays detected by X-ray astronomers must be produced by high-energy particles. It is not surprising, then, that an X-ray image of the sky can look markedly different from an optical image. In essence, X-ray images reveal hot spots in the universe: regions where particles have been energised or raised to very high temperatures by phenomena such as strong magnetic fields or violent explosions. Such conditions exist in an astonishing variety of places, ranging from the vast spaces between galaxies to the bizarre warped space around neutron stars and black holes, in the violent final seconds of a massive star’s normal existence. The X-ray emission from these collapsed stars is billions of times greater than that from the sun. The study of these high-energy processes was defining a new field, high-energy astrophysics, the central problem of which is an understanding of the source of the energy released in X-ray sources.
Chandra: X-ray Astronomy in the New Millennium
During the Uhuru and Einstein observatory project, Giacconi had displayed extraordinary scientific management skills that secured his 1981 appointment as the first Director of the Space Telescope Science Institute, the astronomical research centre on the Homewood campus of Johns Hopkins University in Maryland. Under his leadership, the Institute developed the expertise and capabilities to direct the science mission of the Hubble Space Telescope, the first major optical telescoped to be placed in space, above the distortion of the atmosphere. This gave Giacconi, together with Ethan Schreyer and Rodger Doxsey, the opportunity of transferring some of the X-ray experience to the planning of the Hubble Space Telescope, a project funded by NASA and by the European Space Research Organization (ESRO). Since its launch and deployment in April 1990 Hubble had an unobstructed view of the universe of 38,000 celestial targets and has been used by about 4000 astronomers from all over the world. The mext step will be the Webb Space Telescope, a tennis-court sized telescope orbiting approximately 1 500 000 km beyond the Earth, that will detect infrared radiation, vital for a further understanding of the universe, being able to see many more and much older stars and galaxies than its predecessors Hubble and Spitzer. The project represents an international collaboration of about 17 countries led by NASA, with a planned launch date towards the end of 2010s.
In 1976, even before the launch of Einstein, Giacconi and Harvey Tananbaum had joined in re-proposing a 1.2 m telescope. Unfortunately there was a 20-year hiatus between the proposal and the actual launch of the mission Advanced X-ray astrophysics Facility eventually named Chandra X-ray observatory, in honour of the Indian-American astrophysicist and Nobel Laureate Subrahmanyan Chandrasekhar.
From 1991, Harvey Tananbaum served as Director of the Chandra X-Ray Center at the Smithsonian Astrophysical Observatory in Cambridge, Mass, together with Martin Weisskopf, leading the team that developed, tested and operated the Chandra X-ray Observatory. During that time, from 1993 to 1999, Giacconi served as the director general of the European Southern Observatory (ESO) in Garching, Germany, a scientific leadership role involving ground-based astronomical facilities. In July 1999, Giacconi returned to the United States to become president and chief executive officer of Associated Universities, Inc., the Washington, D.C.-headquartered scientific management corporation operating the National Radio Astronomy Observatory in cooperation with the National Science Foundation. In cooperation with Europe and Japan, the observatory was constructing the world's largest array of millimetre and sub-millimetre radio antennas, called ALMA, in a high and dry site on the Chajnantor plateau at 5,000 meters altitude, in northern Chile. ALMA eventually became fully operational in March 2013.
Chandra was launched on July 23, 1999, after being pursued for more than 20 years as the successor to the Einstein Observatory. The Chandra telescope consists of four pairs of nested mirrors with a 3 nm iridium coating and a high-resolution mirror assembly structure. The highly successful grinding, polishing and coating techniques developed for the Chandra mirrors resulted in the smoothest and cleanest mirrors ever made. The angular resolution of Chandra is astonishing. Since the first pioneering discovery of the first X-ray extra-solar source, the angular resolution improved from 20 to 0.5 arc-seconds and the sensitivity by ten billions. Chandra launched X-ray astronomy into the new millennium. The success of Chandra has been enormous and covers all fields of X-ray astrophysics. This powerful mission was joined in orbit in December 2000 by the European Space Agency's Newton Observatory. Both Chandra and Newton took X-ray astronomy and high-energy astrophysics into a new era. Orbiting at an altitude of up to 139 000 km, Chandra had eight-times greater resolution and is able to detect sources more than 20-times fainter than any previous X-ray telescope. Giacconi himself, who was the principal investigator for the ultra-deep survey with Chandra, was finally able to image at least 90\% of the 0.5 to 6.0 keV background in the Chandra Deep Field South (CDF-S), one of the deepest observations obtained with Chandra, staring at the same path of the sky for 11 days. This region was selected for observation because it has much less galactic gas and dust to obscure distant sources. The final image was the result of the co-addition of 11 individual exposures obtained in 1999-2000, for a total of one million seconds. The source density in the Chandra Deep Field South was about one source per square arc minute. Giacconi used Hubble for optical identifications and VLT for spectroscopy of the sources. The CDF-S became in fact the centre of one of the most comprehensive multi-wavelength campaigns ever carried out with ground-based and space observatories. The CDF-S discovered over 300 X-ray sources, many of them from "low luminosity" Active Galactic Nuclei lying about 9 billion light years away. The study also discovered the then most distant Quasar 2, lying at redshift z=3.7, some 12 billion light years away. A similar survey, the Chandra Deep Field North, was made by observing an area of the sky three-fifths the size of the full moon for 23 days. Even though the faintest sources detected produced only one X-ray photon every four days, Chandra found more than 600 X-ray sources -- most of them supermassive black holes in galaxy centres. By combining the Chandra and Hubble data for this field, astronomers can take a census of the fraction of young galaxies that contain active supermassive black holes back to a time when the universe was only about one billion years old, less than 10% of its present age.
In 2002, Riccardo Giacconi was awarded the Nobel Prize in Physics “for pioneering contributions to astrophysics, which have led to the discovery of cosmic X-ray sources.” He shared the Prize with Raymond Davis and Masatoshi Koshiba who, too, had made pioneering contributions to astrophysics, in particular detecting cosmic neutrinos.
Giacconi's scientific adventure highlighted a new dimension of science: large groups led by a scientist who must figure out worthy scientific goals and at the same time be an expert manager and politician. Confidence in the value of X-ray observations for astronomy was not always widely shared by other scientists and it is hard to convince bureaucrats to direct research funds into new types of observations with unpredictable results. Many observational discoveries in the field of cosmic phenomena were made thanks to technological innovations and were not predicted from theory. The discipline of X-ray astronomy was actually developed largely by physicists with a background in cosmic-ray studies or solar physics, a rich and articulated experience from which they had derived - as Bruno Rossi well emphasized - “a deep-seated faith in the boundless resourcefulness of nature, which so often leaves the most daring imagination of man far behind.”
Bibliography
Clark, G. () Interview with Richard Hirsch. http://www.aip.org/history/ohilist/4555.html#ftn.10
Giacconi R. and Tananbaum H. (1980) The Einstein Observatory: New Perspectives in Astronomy. Science 209 (4459): 865-87
Giacconi R. (2002) Autobiography. Nobelprize.org,
http://www.nobelprize.org/nobel_prizes/physics/laureates/2002/giacconi-bio.html
Giacconi R. (2002) The dawn of X-ray astronomy. Nobel Lecture, Nobel.org
http://www.nobelprize.org/nobel_prizes/physics/laureates/2002/giacconi-lecture.html
Giacconi R. (2005) An education in astronomy. Annual Review of Astronomy and Astrophysics 43(1): 1-30
Giacconi R. (2009) History of X-ray telescopes and astronomy. Experimental Astronomy 25: 143-156
Rossi, B. (1990). Moments in the Life of a Scientist. Cambridge University Press.
Santangelo A. and Madonia R. (2014) Fifty years of X-ray astronomy: A look back and into the (near) future. Astroparticle Physics 53: 130-151