Prof. Dr. Ivar Giaever > Research Profile
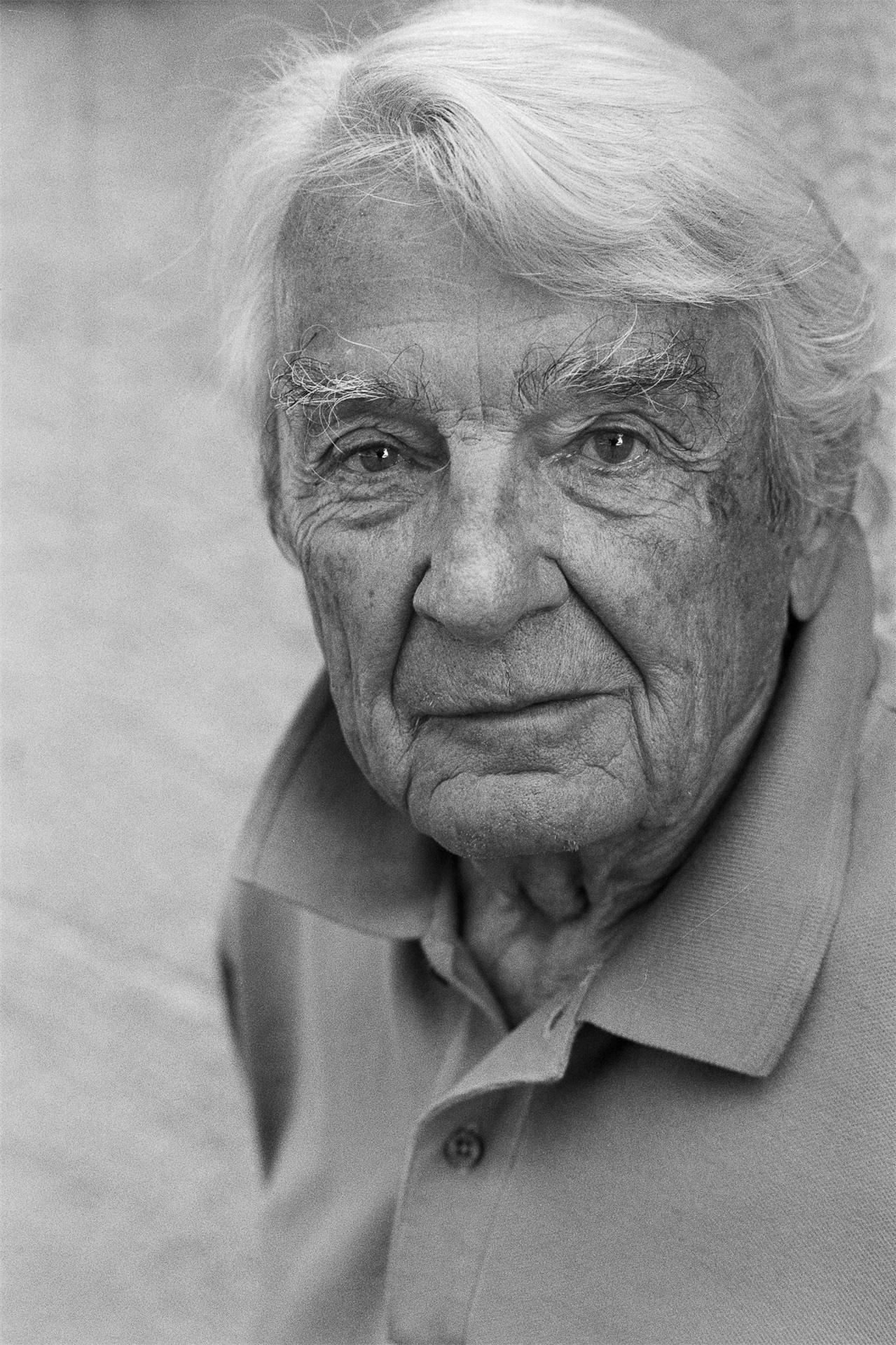
by Roberto Lalli
Ivar Giaever
Nobel Prize in Physics 1973 together with Leo Esaki and Brian D. Josephson "for their experimental discoveries regarding tunneling phenomena in semiconductors and superconductors, respectively".
Training in Engineering
Ivar Giaever was born in Bergen, on the west coast of Norway, on the 5th of April 1929, but grew up in the Toten district in East Norway and received his secondary education in the nearby city of Hamar. After a one-year working experience at the Raufoss Ammunition Factories, in 1948 he enrolled at the Norwegian Institute of Technology in Trondheim to study mechanical engineering, in which he graduated in 1952.
In 1954, Giaever emigrated to Canada where he shortly joined the Advanced Engineering Programme of the Canada division of General Electric—a graduate level course in engineering and applied mathematics established by the General Electric Company to train its members. In 1956, he emigrated again to complete the A, B and C engineering courses at General Electric in Schenectady, New York. After completing his education, Giaever began to do pure research working at the General Electric Research and Development Center in 1958, at the same time entering the Rensselaer Polytechnic Institute to do graduate work in physics.
Tunnelling in Superconductors
As his first research commitment, he was assigned to focus on thin film technology. Working with John C. Fisher—a mechanical engineer with a strong interest in theoretical physics—Giaever began investigating the possible applications of thin films in electronic devices. They focused on the tunnelling effect that might occur when two conductors were separated by a very narrow film.
Quantum tunnelling—a quantum mechanical effect according to which particles have a finite probability to penetrate and pass through an energy barrier—was one of the most striking effects that differentiate the predictions of quantum mechanics from those of Newtonian mechanics. The phenomenon was a consequence of wave-particle duality—first formalized by de Broglie in 1923—and its elaboration in the Schrödinger equation of wave mechanics. In 1927, the hypothesis that electrons have wavelike properties had received persuasive empirical confirmation thanks to the observations of the diffraction of electron beams by C. Davisson and L. Germer at the AT&T Bell Telephone Laboratories (Bell Labs) and by G. P. Thomson at the University of Aberdeen.
The astonishing implications of the new conception of matter were rapidly explored and quantum tunnelling became a possible explanation of until then puzzling phenomena. One of the early employments of the still undefined tunnelling concept was in nuclear physics, when G. Gamow, and, independently, R. Gurney and E. Condon interpreted the alpha decay as the quantum tunnelling of the alpha particles through the nuclear potential barrier. When Gamow was putting forward his ideas, he was pursuing his doctorate studies at the University of Göttingen—one of the major research centres involved in the development of quantum mechanics. There, Max Born had established a research program in which a large number of talented younger researchers were exploring the mathematical structure of the new theory as well as its physical implications. After having clarified some features of the theory, M. Born recognized the relevance of Gamow’s interpretation of the alpha decay and understood that quantum tunnelling was a general physical property of quantum mechanics that had to be observable in other physical systems. However, while the tunnelling concept was extremely useful in the field of nuclear physics, at first it did not meet the same success when it was employed in the physics of solids. The theoretical models developed in the 1930s and 1940s did not correctly represent the empirical facts observed in conduction across solid junctions.
In 1947, J. Bardeen, W. Brattain, and W. Shockley discovered the transistor at Bell Labs. This discovery led to a revolution in the field of electronic communication, which also implied that semiconductor devices began to receive much stronger attention because of their important technological applications. Moreover, the discovery of the transistor itself was made possible by the fact that the manufacture of doped semiconductors with well-defined features had enormously improved during World War II. Further technological improvements in the doping of semiconductors resulted in the first important discovery in the field of quantum tunnelling in solids. Working at the Sony Corporation, the Japanese physicist Leo Esaki observed clear evidence of quantum tunnelling in highly doped p-n junction. These observations resulted in the invention of the Esaki diode, or tunnel diode, which the Sony Corporation began manufacturing in 1957, even before Esaki had made his findings public.
In his Nobel lecture, Giaever confessed that he was not aware of all these developments. Nor had he much experience in experimental physics. However, in 1958 Giaever and Fisher made rapid progress thanks to their strong training in mechanical engineering. After some failures they decided to employ evaporated metal films and to separate them with a naturally grown oxide layer. The chosen manufacturing procedure allowed them to observe convincing evidence of tunnelling in their metal-oxide-metal junctions. In spite of their precise observations, definitive evidence was still lacking that the current detected was due to tunnelling and not to other mechanisms, such as breaks in the insulating layer.
Giaever then got interested in the phenomenon of superconductivity and in the BCS theory. In 1957, J. Bardeen, L. Cooper, and J. Schrieffer had put forward a microscopic theory that predicted the phenomenological properties of superconductivity starting from first principles of quantum mechanics. As other phenomenological theories of superconductivity proposed earlier, the BCS theory implied an energy gap in the spectrum of possible energy states. In the BCS theory, the superconducting ground state of Cooper pairs was separated by this gap from the first quasi-particle excitation.
Giaever hypothesised that the superconducting energy gap would have testable consequences on the tunnelling of electrons between superconductors separated by an insulating layer. While in the tunnelling of electrons between two metals separated by an insulating film the current was proportional to the applied voltage, in a metal-insulator-superconductor junction the energy gap hypothesis implied that the relationship between the electric current and the voltage was drastically altered.
Giaever’s expertise in the quantum tunnelling in metal-insulator-metal junctions led him to realize that the experiment could be successfully performed once he had a way to cool one of the two metals below its critical temperature. With the support of many colleagues at the General Electric Laboratory, which helped him with the theoretical work and provided him with the necessary instruments, in 1959 Giaever was able to carry out his experiments employing lead (whose critical temperature is 7,2 K) and aluminium (whose critical temperature is much lower, at 1,2 K) separated by oxide layers. The result was that once he was able to produce an oxide layer sufficiently narrow (30 Å,) and as soon as the lead changed from the normal to the superconducting state, the current-voltage characteristic markedly changed in just the way expected from the superconducting energy gap hypothesis. To confirm that the effect he was observing was due to the energy gaps of superconductors, Giaever applied different magnetic fields to its apparatus because sufficiently strong magnetic fields destroy the superconducting state. Again, the experiment was successful in that for magnetic fields greater than 2400 gauss the current-voltage characteristic became linear, that is equal to that observed in the metal-oxide-metal junction.
As a second step, in 1960 Giaever also performed an experiment on two different superconductors separated by a thin oxide layer. Even in this case, the result was as expected. Once the second metal also reached the superconducting state the resistance became negative. Giaever published his discoveries in two short articles, appearing in the journal Physical Review Letters in August and November 1960, respectively. Giaever’s findings had an enormous impact not only because he provided a very direct evidence of the energy gap in favour of the BCS theory, but also because it soon served as one of the sources of inspiration for the theoretical work undertaken by the British theoretical physicist Brian Josephson two years later. By looking at the quantum mechanical description of superconductor-insulator-superconductor junctions implied by some formulations of the BCS theory, Josephson predicted that the tunnelling of single electrons was not the only tunnelling effect to be expected. His calculations showed that under some circumstances Cooper pairs should be able to pass through the potential barrier as well—a phenomenon now called Josephson effect. Shortly after the Josephson effect was predicted, convincing evidence was provided that this was indeed the case.
In the meantime, Giaever was continuing to explore the potentiality of tunnelling for investigating the properties of superconductors with other collaborators at the General Electric Laboratory reaching a very high level of experimental precision. In 1962, Josephson predicted two effects related to the tunnelling of supercurrents: 1) the DC Josephson effect in which a direct supercurrent flew through a barrier potential without any applied external potential; and 2) the AC Josephson effect, according to which high frequency alternating supercurrents flew through the barrier when a certain voltage was applied, the frequency of which was independent of the properties of the superconductors and depended instead on universal constants through the formula 2eV/h (where e is the charge of the electron, h is the Planck constant, and V is the bias potential). Once Giaever became aware of Josephson’s predictions, he understood that he had indeed observed some clear examples of the DC Josephson effect in his previous experiments with two superconductors, but had misinterpreted them as due to breaks in the oxide layer.
In 1965, Giaever made his contribution to the widespread acceptance of the reality of the Josephson effect by detecting AC Josephson tunnelling. By that time, the Josephson effect had already become one of the most important macroscopic and technologically applicable effects of first principles of quantum mechanics. Giaever’s contributions to the discovery and application of tunnelling in solids was awarded with the Nobel Prize in Physics in 1973—the year after Bardeen, Cooper and Schrieffer had received the award for the discovery of the BCS theory. Giaever divided one half of the Nobel Prize with Leo Esaki “for their experimental discoveries regarding tunnelling phenomena in semiconductors and superconductors, respectively.” The other half went to Josephson “for his theoretical predictions of the properties of a supercurrent through a tunnel barrier, in particular those phenomena which are generally known as the Josephson effects.”
From Solid-state Physics to Biophysics
Giaever’s research on tunnelling in superconductors was part of his dissertation that allowed him to receive his PhD from the Rensselaer Polytechnic Institute in 1964. After earning the PhD, Giaever continued to work on tunnelling technology at the General Electric until 1969, when he won a Guggenheim Fellowship to spend one academic year at Cambridge University to study biophysics. After his return to General Electric, he applied his expertise on the quantum theory of solids and its technological application to study the behaviour of protein molecules at solid surfaces.
In the following year, Giaever continued to perform research in biophysics, making observations on the motion and growth of mammalian cells in tissue culture by measuring the electromagnetic properties of normal and cancerous cells with a small electrode. His work was aimed at developing a method for easily distinguishing between normal and cancerous cells that could be an improvement with respect to those methods that employed optical microscopy alone. The second line of research concerned the study of the kinds of surfaces to which the tumoral cells can be attached.
Giaever continued to do research on biophysics at General Electric till 1988, when he left the industrial environment to accept a professorship at the Rensselaer Polytechnic Institute—the institution at which he had earned his PhD more than 20 years earlier. This change was motivated by the decision of the General Electric management not to pursue basic research in its laboratories any longer. This state of affairs did not only lead Giaever to go into an academic career, but also convinced him that he should found his own small high-tech enterprise in order to find sufficient financial support to do basic research in the fields he was investigating. In 1991, Giaever and his associate C. R. Keese formed the company Applied BioPhysics Inc. to develop and commercialize an impedance method for the monitoring of animal cells in tissue culture called the Electric Cell-substrate Impedance Sensing. The company is still very active in providing instruments and arrays for the investigation of cell growths.
Unorthodox views in the climate change controversy
Giaever’s name has recently made a comeback in public debates for he is one of the few authoritative scientists to maintain a strong sceptical position against the overwhelming consensus on the man-made global warming and the related campaign to take immediate actions to avoid its potentially catastrophic consequences to the environment and its inhabitants. Giaever repeatedly voiced his doubts on the reliability of the scientific methodology behind claims that there is conclusive evidence for global warming and he stated that, even if there is such a thing as a century-long steady increase of the average temperature of the Earth, this change need not be primarily caused by human activities. He has strongly criticized the scientific communities for their position on such claims by stating that global warming had become a sort of religion, while climate change has, in his opinion, the status of a pseudoscience.
These views led to his resignation as a member of the American Physical Society (APS) in 2011 in order to protest against a policy statement of the APS in which it was declared: “Emissions of greenhouse gases from human activities are changing the atmosphere in ways that affect the Earth's climate. They are emitted from fossil fuel combustion and a range of industrial and agricultural processes. The evidence is incontrovertible: global warming is occurring. If no mitigating actions are taken, significant disruptions in the Earth's physical and ecological systems, social systems, security and human health are likely to occur. We must reduce emissions of greenhouse gases beginning now.” Giaever was particularly disturbed by the employment of the word ‘incontrovertible’ in this statement, because according to him the scientific approach should always leave space to doubts and every statement on the physical world should always be critically analysed as well as tested again and again.
While in general terms, Giaever’s discourse can be considered reasonable, the employment of such arguments on a topic with such potentially devastating consequences for human life has been strongly criticized and is not shared by the great majority of scientists. Earth science experts have criticized him on the grounds that Giaever was employing arguments that had long been debunked in the scientific debate on climate change and that he did not know the field well enough to understand the data he is trying to interpret. It has been recently shown that there is an overwhelming consensus among climate scientists that global warming of unprecedented speed is occurring and that it is caused by human activities. A survey showed than more than 97% of peer-reviewed papers addressing climate change between 1991 and 2011 endorse this view. This consensus is based on a series of common principles and practices that constitute a scientific discipline, of which Giaever has no direct knowledge for he has never worked seriously on it.
Moreover, the debate on climate change has always had strong political and economic implications, for most scientists are requiring the implementation of immediate international policies in order to reduce the emission of greenhouse gases, regarded as centrally responsible for the rapid global warming. Any criticism and doubt rose against the scientific consensus is often interpreted as a possible motivation to delay or interrupt action most scientists consider as necessary. In this sense, the view of authoritative scientists, experts in other fields, is often considered among the most dangerous rhetorical weapons to create doubts in the uninformed population.
Giaever’s position is mainly motivated by his strong and long-lasting belief in the power of technological advancements to create the best condition for the thriving of humanity. Only through such technological advancements, his argument goes, human beings have been able, and will be able, to overcome the possible destructive forces of nature. This belief has characterized his career at the boundary between research in theoretical physics and application in engineering and biophysics.
Bibliography
Esaki, L. (1973) Nobel Lecture: Long Journey into Tunnelling. In Stig Lundqvist (eds.) (1992) Nobel Lectures, Physics 1971-1980. World Scientific Publishing Co., Singapore, pp. 126-133.
Giaever I. (1973) Nobel Lecture: Electron Tunneling and Superconductivity. In Stig Lundqvist (eds.) (1992) Nobel Lectures, Physics 1971-1980. World Scientific Publishing Co., Singapore, pp. 137-153.
Hoddeson, L., Braun, E., Teichmann, J., & Weart, S. (eds.) (1991) Out of the Crystal Maze: Chapters from the History of Solid-State Physics. Oxford University Press, New York.
Ivar Giaever - Biographical. Nobelprize.org. Nobel Media AB 2014. Retrieved 16 January 2015. http://www.nobelprize.org/nobel_prizes/physics/laureates/1973/giaever-bio.html
Josephson, B. D. (1973) Nobel Lecture: The Discovery of Tunnelling Supercurrents. In Stig Lundqvist (eds.) (1992) Nobel Lectures, Physics 1971-1980. World Scientific Publishing Co., Singapore, pp. 157-164.
Press Release: The 1973 Nobel Prize in Physics. Nobelprize.org. Nobel Media AB 2014. Retrieved 10 January 2015. http://www.nobelprize.org/nobel_prizes/physics/laureates/1973/press.html