Prof. Dr. Sheldon Lee Glashow > Research Profile
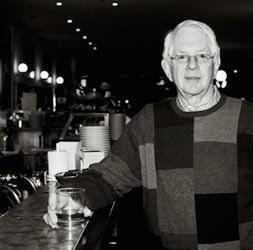
by Roberto Lalli
Sheldon Lee Glashow
Nobel Prize in Physics 1979 together with Abdus Salam and Steven Weinberg
"for their contributions to the theory of the unified weak and electromagnetic interaction between elementary particles, including, inter alia, the prediction of the weak neutral current".
Sheldon Lee Glashow is an American theoretical physicist who has made major contributions to the construction of the Standard Model of particle physics. Ever since Maxwell had unified - in the 1860s - apparently distinct phenomena such as electricity, magnetism and light in a single theory, one of the central aspirations of theoretical physics has been to elaborate a theoretical scheme that explains as many as possible natural phenomena starting from few elementary concepts. Currently, the Standard Model represents the main result of this program since it allows for a unified description of three of the four fundamental interactions of nature: electromagnetic, weak and strong interactions. Glashow has been committed to this unification program since his formative years - a period in which the difficulties concerning the search for a simple and elegant theory embracing the various interactions were discouraging. In 1961, he proposed a symmetry structure for the unification of electromagnetism and weak interactions in a unique mathematical formalism - the SU(2)xU(1) gauge group. Glashow’s model implied the existence of four force carriers: the photon carrying electromagnetic force and three vector bosons mediating weak interactions. Following theoretical developments as well as extremely precise experimental confirmations, the spontaneously broken SU(2)xU(1) structure has become the basis of the currently accepted electroweak theory. After this theory gained consensus within the physics community, Glashow was awarded the 1979 Nobel Prize in Physics, along with Steven Weinberg and Abdus Salam. Apart from his Nobel–winning theory, Glashow has been producing a number of works that have enhanced our understanding of particle interactions. Among the most influential contributions, there are Glashow’s publications leading to the prediction and experimental discovery of the charm quark. Not only was Glashow one of the first theoreticians to predict its existence, but he also discovered, along with his collaborators John Iliopoulos and Luciano Maiani, a phenomenological mechanism that explains some selections rules of the weak interactions as well as the absence of flavour changing neutral currents. The Glashow-Iliopoulos-Maiani (GIM) mechanism requires the existence of the charm quark, and Glashow dedicated several efforts to explore theoretically the behaviour of charmed hadrons, i.e., those particles that have at least one charm quark among their constituents. Following theoretical predictions made by Glashow and others, experimental physicists were able to detect the charmed particles in the 1970s, leading to a further confirmation of the validity of the Standard Model.
Towards the Unification of Electromagnetic and Weak Interactions
Glashow was born in New York City on December 5, 1932. He was the last of three children of Lewis Glashow and Bella née Rubin. Both his parents were Jewish immigrants that left czarist Russia at the beginning of the 20th century. Glashow showed an early inclination for natural sciences, which his parents encouraged. He attended the Bronx High School of Science, where he had as classmate Steven Weinberg, with whom he would share the Nobel Prize some thirty years later. Glashow and Weinberg also shared the undergraduate path at Cornell University, where Glashow earned his Bachelor’s degree in 1954. After graduation, he pursued his studies at Harvard University. There, Glashow obtained his Master’s degree in 1955, and began his PhD studies under the supervision of Julian Schwinger who would become a co-recipient of the 1965 Nobel Prize in Physics for his foundational work concerning the renormalization of quantum electrodynamics.
Glashow’s training under Schwinger was essential in paving the way for the work Glashow made to uncover the symmetry structure of the electroweak interactions.
In 1957, Schwinger proposed that the unification of weak and electromagnetic interactions should be based on quantum field theory, especially on the non-Abelian Yang-Mills gauge theory developed in 1954. Schwinger’s belief in the electroweak synthesis stemmed from the fact that a gauge field theory of weak interactions alone was not renormalizable because of the dimension of the Fermi coupling constant giving the strength of weak interactions. Schwinger advised Glashow to explore possible solutions to this problem, which Glashow did in his dissertation “The Vector Meson in Elementary Particle Decays.” Sharing Schwinger’s enthusiasm for the unification project, Glashow devised a mechanism through which three vector bosons mediated the electroweak forces: two charged heavy bosons carrying the weak force (called W+ and W-), and a neutral massless boson mediating the electromagnetic force (the photon γ). These spin-1 particles were thought to form a triplet of the symmetry group SU(2). Although promising, such a unification scheme presented several theoretical problems. The most disturbing was that photons conserved parity and strangeness, while the two charged heavy bosons Ws did not. Some physicists, including Glashow, soon recognized that it seemed hopeless to build a theory of electroweak interactions that also accounted for parity violation through the SU(2) scheme.
After having obtained his PhD in 1959, Glashow went to the Institute for Theoretical Physics of the University of Copenhagen to do postdoctoral research. Glashow’s plan was to continue on to Russia in order to work with Soviet Nobel laureate Igor Tamm, but his visa never arrived. As a consequence, he spent the entire postdoc fellowship in Copenhagen. Later, he considered himself fortunate that the events went that way. During this period, indeed, he completed his Nobel-winning model published in 1961 with the title “Partial-Symmetries of Weak Interactions”. Glashow hypothesized that the symmetry structure underlying the electroweak unification was larger than SU(2). The gauge symmetry group, Glashow proposed, was SU(2)xU(1), which required four different force carriers. Of these, two were heavy charged bosons mediating charge-changing currents of weak interactions. The other two bosons were neutral and their linear combinations led to the prediction of two different particles with definite masses: the massless photon mediating the electromagnetic force and the neutral heavy boson carrying weak neutral-current interactions – the particle now called Z. Glashow’s model provided a coherent picture of the electroweak unification in gauge field theory formalism, but had some relevant shortcomings.
From a theoretical perspective, the mass differences between the three heavy bosons and the massless photon remained without a convincing explanation. In the early 1960s, some studies on the Yang-Mills theory showed that the gauge bosons should remain massless, while bosons mediating the weak force were necessarily massive in order to explain the short range of these interactions. For this reason, the model Glashow proposed was unsatisfying. From the experimental point of view, Glashow’s theory predicted the existence of weak neutral currents (in the interactions mediated by the Z boson), which had never been observed. In addition, Glashow’s model regarded leptons alone and did not include hadrons, which also interact weakly. In the following years, all these shortcomings were removed thanks to the efforts of many physicists. Starting from 1963, a number of theoreticians put forward a mechanism that allows gauge vector bosons to acquire their mass through the spontaneous breaking of the underlying gauge symmetry (the so-called Higgs mechanism). In 1967-68, Steven Weinberg and Abdus Salam both provided a full-fledged theory of electroweak interactions of leptons based on the SU(2)xU(1) gauge symmetry by employing the Higgs mechanism to explain the masses of three of the four spin-1 gauge bosons (the Ws and the Z), the fourth being the massless photon. Although the theory proposed independently and simultaneously by Weinberg and Salam was internally coherent, it was rarely quoted in the following few years. Commentators agree that the main reason why the Weinberg-Salam theory went unnoticed was that it was not proved to be renormalizable. In 1971, Dutch theoretical physicist Gerardus ‘t Hooft - working with his thesis advisor Martinus J. G. Veltman - provided this missing proof. After this and subsequent works demonstrating the renormalization of the Glashow-Weinberg-Salam theory, such a theory became a leading candidate for the explanation of electroweak interactions although it still lacked an empirical confirmation. It came in 1973, when experiments conducted at CERN showed that weak neutral currents do exist. Following this early confirmation, other experiments followed. Particularly relevant was the discovery of weak neutral-current processes concerning charged leptons in 1978, which convinced many physicists that the SU(2)xU(1) electroweak unification was correct. The following year, the Nobel Prize in Physics was conferred to Glashow, Salam and Weinberg “for their contributions to the theory of the unified weak and electromagnetic interaction between elementary particles, including, inter alia, the prediction of the weak neutral current.” Only four years later – in 1983 - the vector W and Z bosons themselves were eventually discovered at the Super Proton Synchrotron at CERN, which was specially converted to a proton-antiproton collider. Two among the main experimental researchers who contributed to the discovery of the electroweak gauge bosons were awarded the Nobel Prize the year after the first positive results were published. In 1984, Carlo Rubbia and Simon van der Meer shared the Nobel Prize in Physics “for their decisive contributions to the large project, which led to the discovery of the field particles W and Z, communicators of weak interaction.”
Beyond the Three-Quark Model
In the 1960s, one of the main problems affecting the theoretical descriptions of weak interactions was that these interactions involve leptons, of which four were known at the time (electron, muon and their corresponding neutrinos), and hadrons in a similar manner. To provide an analogy between quarks and leptons, several theoretical physicists began suggesting the existence of four quarks instead of the three hypothesized by Gell-Mann in 1964. In the very same year, Glashow, along with J. Bjorken, christened this hypothesized fourth quark “charmed,” claiming that it should be heavier than the u-quark, but have the same electric charge + 2/3.
The various suggestions of the mid-1960s were not developed until Glashow, Iliopoulos and Maiani published the foundational paper “Weak Interactions with Lepton–Hadron Symmetry” in 1970. The three authors put forward a phenomenological model for the weak interactions based on four basic quark fields, which was successful in explaining several observations concerning hadronic weak interactions. While preserving the strong interactions’ symmetries, the GIM model allowed for an explanation of why the strangeness-changing neutral currents were suppressed in all order of perturbation theory as well as for a derivation of the weak interactions’ selection rules. At the end of the paper, Glashow, Iliopoulos and Maiani also discussed the possible experimental procedures that could demonstrate the existence of charmed particles. Like strangeness, the charm quantum number was conserved in strong interactions, but violated in weak interactions. The difficulties in observing a charmed particle came from the very short life time of these kinds of particles. By applying the GIM model, however, it was possible to calculate the mass of the charm quark. Such a calculation guided the following studies for experimental observations. Several researchers began conceiving methods to experimentally discover the predicted charmed particles. After four years, two different groups headed, respectively, by Burton Richter and Samuel C. Ting observed simultaneously a particle that was soon interpreted correctly as a charmed meson with a mass of about 3.1 Gev. Richter’s team operated at the Stanford Linear Accelerator Center (SLAC) and christened the particle Psi, while Ting’s group worked at the Brookhaven National Laboratory and named the particle J. Since the two groups gave two different names to the same particle, this charmed meson has been called J/Psi ever since. Although several physicists soon suggested an interpretation of the J/Psi particle based on the charm quark hypothesis, it took a couple of years to build consensus on this interpretation because it was necessary to add further hypotheses to explain the totality of acquired data. In 1976, the charm quark hypothesis and the consequent interpretation of the J/Psi particle as the ground state of the charm quark and its antiquark were finally established. The same year both Richter and Ting were awarded the Nobel Prize in Physics “for their pioneering work in the discovery of a heavy elementary particle of a new kind.”
The First Grand Unified Theory
Glashow did not diminish his efforts to find a simple theory for the unification of particle interactions after the establishment of the Standard Model had vindicated his early attempts. In his Nobel lecture, Glashow made it clear that he did not believe that the Standard Model would “long survive as a correct and complete picture of physics.” Already in 1973, along with Howard Georgi, Glashow put forward the first Grand Unified Theory (GUT) whose aim was to find a larger symmetry structure for the unification of electromagnetic, weak and strong interactions that contains the Standard Model at low energies. At high energies, Glashow and Georgi hypothesized, all these interactions were unified under the gauge group SU(5) and a single coupling constant. The SU(5) scheme predicted that the proton decays and, consequently, calculations of proton’s lifetime were made. These predictions do not agree with the lower limit for the lifetime of the proton derived from observations. In spite of this empirical issue, the numerous subsequent Grand Unified Theories were based on that of Glashow-Georgi because of its elegance and simplicity. Up to now, there is no GUT that has gained universal acceptance within the community of physicists, but this research field of theoretical physics is still very much alive, and some believe that the difficulties such theories have been encountering will be overcome in the near future.
Bibliography
Fitch V. L., & Rosner L. (1995) Elementary Particle Physics in the Second Half of the Twentieth Century. In Brown, L., Pippard, B., & Pais, A. (Eds.). Twentieth Century Physics (Vol. 2). AIP, New York, pp. 635-794.
Glashow, S. L. (1980). Towards a unified theory: Threads in a tapestry. Reviews of Modern Physics 52: 539-543.
Glashow, S. L. (1979) Sheldon Glashow – Biographical. Available at http://www.nobelprize.org/nobel_prizes/physics/laureates/1979/glashow-bio.html.
Hoddeson, L., Brown, L. M., Riordan, M. & Dresden, M. (1995) The Rise of the Standard Model: Particle Physics in the 1960s and the 1970s. Cambridge University Press, Cambridge.
Pais, A. (1986) Inward Bound: Of Matter and Forces in the Physical World. Clarendon Press, Oxford.