Prof. Dr. Maria Goeppert Mayer > Research Profile
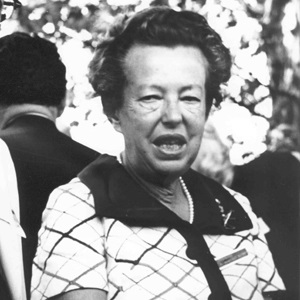
by Luisa Bonolis
Maria Goeppert Mayer
Nobel Prize in Physics 1963
together with Eugene Wigner and J. Hans D. Jensen "for their discoveries concerning nuclear shell structure".
Growing up in Göttingen
Maria Gertrude Käte Goeppert was the only child of well-educated upper-middle-class parents. She was born on July 28, 1906, in what was then the German city of Kattowitz in Upper Silesia, and is now the Polish city of Katowice. Her father Friedrich Goeppert, a professor of medicine at the university, who came from a long line of scientists, always encouraged his daughter's natural curiosity and adventurousness, taking her on science walks, hunting for quarry fossils and showing her eclipses of the sun and the moon. In 1910, when she was four, the family moved to the small university town of Göttingen, where they had the great mathematician David Hilbert as a neighbour and friend, and their social circle included many of the scientists of the great university. As a Germany university professor, her father occupied a position of great prestige and when Maria grew older there was never any doubt in her mind that she would study at the university. Both the town and its university profoundly influenced her life and dominated the whole structure of her education.
In 1924, after taking the so called Abitur a year earlier than normal, she entered the Georgia Augusta University to study mathematics, for which she had a natural talent. At that time fewer than one in ten German university students was a woman. The Georgia Augusta had long been famous for mathematics and was becoming so for theoretical physics as well. In 1927, inspired by the lectures of Max Born, one of the founding fathers of the new quantum theory, Maria Goeppert switched to physics: “Mathematics began to seem too much like puzzle-solving... Physics is puzzle-solving, too, but of puzzles created by nature, not by the mind of man... Physics was the challenge.” Furthermore, she recalled that “quantum mechanics was young and exciting.” It was just at this time that the great developments in quantum mechanics were taking place, with Göttingen as one of the principal centres. Born's advanced theory seminar attracted the most brilliant gathering of young talents of the time. Among its stars were Paul Dirac, Wolfgang Pauli, Werner Heisenberg, Robert Oppenheimer from the United States, Enrico Fermi from Italy, and Eugene Wigner and John von Neumann from Hungary. Many of them were future Nobel Prize winners. Maria Goeppert's closest friends were fellow students Victor Weisskopf and Max Delbrück; the latter became Lise Meitner's assistant in Berlin and later became a leading molecular biologist. Other friends who became science luminaries were Linus Pauling, Leo Szilard, and Arthur Holly Compton. She attended Göttingen at the peak of its prestige in mathematics and physics, and became the personal friend of many of its leading stars. Attracted by Born and by the leading physicist James Franck, who had just been awarded the 1925 Nobel Prize for physics, most of the generation who developed quantum mechanics during the mid-1920s and early 1930s visited Göttingen. She was friendly with many of them and, as a result, was among the first practitioners of the new physics. In describing the behaviour of atoms, nuclei and their components, quantum mechanics was uniting physics, chemistry, astronomy, and much of biology. It was developed under the loose leadership of Niels Bohr in Copenhagen by a small group of Europeans, particularly Maria's teacher Max Born and Werner Heisenberg in Göttingen, Erwin Schrödinger and Wolfgang Pauli in Austria, and Paul Dirac in England. As a student of Max Born, a theoretical physicist with a strong foundation in mathematics, Maria was well trained in the mathematical concepts required to understand quantum mechanics. This and her mathematics education gave her early style of research a strong mathematical flavour, yet James Franck's non-mathematical approach to physics also had a great influence at this stage of her work.
In 1927 her beloved father died unexpectedly. Young Maria was unprepared for this blow. Nevertheless, she resolved to complete her doctorate knowing that this would be the best way to honour his memory.
Like most other residents of Göttingen, her widowed mother began taking young people as boarders in her house, for the University provided no housing for students. One of them was a post-doctoral student in chemistry from California named Joseph Mayer, who had come to Göttingen to study quantum mechanics with James Franck, attracted by Göttingen's growing reputation as a centre for the study of quantum mechanics, that was just beginning to be applied to chemistry. Joe Mayer had graduated from the California Institute of Technology and had earned his Ph.D. at the University of California at Berkeley, he could argue with verve and style about almost anything. According to Born, Maria was “pretty, elegant, a high-spirited lady traveling in the best Göttingen style”. Joe and Maria became great friends and in January 1930 they married. In March, she completed her doctoral dissertation 'On Elemental Processes with Two Quantum Jumps', which many years later was described by Eugene Wigner as a "masterpiece of clarity and concreteness." It was a theoretical calculation of the probability that an electron orbiting an atom's nucleus would emit not one, but two, photons as it jumps to an orbit closer to the nucleus. It was actually the first theoretical study of the phenomenon of double quantum emission, that she later used to determine the probability of double beta decay. This process was discovered a few years later, in planetary nebula, and it plays an important role in lasers today. Her solution was confirmed by the laser physics of the 1960s and is still quoted in papers on optics, atomic physics, and molecular physics. She also contributed an eight-page section to a quantum mechanics book that Born wrote with Pascual Jordan. Maria Goeppert, now Maria Mayer, had in Göttingen only first-class mentors and friends, and everybody was enthusiastic about her.
A New Homeland
Meanwhile, her husband had obtained a position as assistant professor of chemistry at Johns Hopkins University and so the couple moved to Baltimore. When Maria Goeppert Mayer started on her scientific career, prejudice against women scientists was so widespread that, until at least the 1920s, a woman who chose a life in physics practically had to renounce the possibility of marriage and motherhood. After her marriage, Maria had thus become one of the first women to attempt balancing family life and physics. To her great dismay - and despite her impressive academic credentials - at that time universities were rather hesitant to hire women for academic positions. Moreover, in America many universities had 'anti-nepotism' rules, preventing husband and wife both holding academic positions in the same institutions. There was thus no chance of an academic post for her there. However, she was assigned to help a member of the physics department with German correspondence, given a small salary and an office, and permitted to participate in the university's scientific activities. Since no-one was working on quantum mechanics at Johns Hopkins, on Born's recommendation she collaborated in research with the Vienna born physicist Karl Herzfeld, who was in the vanguard of chemical physics, a very new field of study at the time. Her background in quantum mechanics was far superior to that of everyone else at Hopkins and this gave her an unprecedented opportunity. In addition, her knowledge of chemistry set her apart from many other theoretical physicists of the time, who took great pride in not knowing one chemical compound from another. Goeppert Mayer published some papers with Herzfeld and with her husband, involving the application of the new field of quantum mechanics to problems in physical chemistry. Quantum mechanics was just beginning to have a profound effect on chemistry. She became involved in pioneering work on the structure of organic compounds with a student of Herzfeld's, A. L. Sklar, and in that work she applied her special mathematical background, using the methods of group theory and matrix mechanics. Their paper on the spectrum of benzene, a most important organic molecule, was a real milestone in quantum chemistry. During the summers of 1931, 1932, and 1933, she returned to Göttingen, where she wrote several papers with Max Born on beta decay.
But in 1933, when Goeppert Mayer became a naturalized American citizen, Hitler came to power in Germany. The result of the first racial laws was that some 200 Jewish teachers and professors were removed from their posts at Göttingen and sent out of Germany. Her beloved friends Born and Franck were among the dozens of physicists and mathematicians who left Germany almost immediately. At that time, the Mayers opened their large Baltimore house to a seemingly endless stream of German exiles, most of them Jewish refugees. Franck's presence at Baltimore turned Hopkins into a world centre for atomic physics.
At Johns Hopkins, Goeppert Mayer was simply tolerated; still, it gave her access to the university facilities, provided her with a place to work in the physics building, and encouraged her to participate in the scientific activities of the university. In the later years, she earned the opportunity to present some lecture courses for graduate students and at the time she became John Wheeler's favourite professor. Although she taught some of the most difficult and complicated courses at John Hopkins, Maria never received a professor's salary. She also collaborated with her husband on a textbook, Statistical Mechanics. When the book, which became a classic, was published in 1940, Joseph Mayer was appointed to a much-better paid position as associate professor of chemistry at Columbia University and so they moved to New York. Unfortunately, it was assumed that her husband had done most of the work on the book, so she did not receive her due credit and Columbia offered her even less official recognition than she had received at Johns Hopkins. Although she was provided with an office, she received neither an appointment nor a salary. Nevertheless, she worked at Columbia with Enrico Fermi and Harold C. Urey on chemical and atomic structure, and Urey, who had a great respect for Maria as a scientist, found a place for her in the chemistry department, giving her the title of lecturer in chemistry. It was the beginning of a close relationship between the Mayers and the Ureys, which was to continue throughout Maria's life. It was at Columbia, too, that she first began to come under the influence of Enrico Fermi, who suggested that she attempt to predict the valence-shell structure of the transuranium elements. By making use of the very simple Fermi-Thomas model of the electronic structure of the atom, she came to the conclusion that these elements would form a new chemical rare-earth series. Her oversimplified model subsequently turned out to be a remarkably accurate prediction of their qualitative chemical behaviour. Neptunium and plutonium were discovered in 1940 and Goeppert Mayer was the first person to work out the atomic properties of these transuranic elements, which have interesting similarities to the rare earths.
In 1941, after twelve years in the profession, Mayer eventually received her first paid teaching appointment, a part-time position at Sarah Lawrence College. The following year, when Urey brought her into the Manhattan Project, the massive effort to develop the atomic bomb, she headed a team that investigated the possibility of separating fissionable uranium-235, one of the three naturally occurring forms of uranium, from the much more abundant uranium-238 by photochemical reactions. She had only two or three scientists working under her at the start, but she ended up with over fifty. In 1945, she also worked at Los Alamos Laboratory with the brilliant Hungarian physicist Edward Teller, with whom she co-authored an important paper on the origin of elements, and from whom she learned about the latest developments in theoretical physics, especially nuclear physics. Teller later became known as "the father of the hydrogen bomb", but his interest in furthering this technology eventually brought about his alienation from most of the scientific community.
The war in Europe came to an end in the spring of 1945, and by this time, like many others scientists, Goeppert Mayer had come to hate being part of the team that had made possible the creation of the bomb that was just waiting to be tested. The request made by many scientists, asking that the demonstration of the new weapon take place on an uninhabited island to prove its might to the Japanese government, was not granted. The U.S. government and military and many other scientists were convinced that the bomb had to be dropped - to spare the American lives that would surely be lost in a ground invasion of Japan. The nuclear bombing of the two Japanese cities of Hiroshima and Nagasaki, effectively ended the Japanese participation in World War II, but, actually, according to historians, could be seen as the first act of the Cold War that would dominate international relations throughout the world for about forty years.
Magic Numbers
After the war, Joseph Mayer was appointed Professor in both the Chemistry Department and the newly formed Institute for Nuclear Studies of the University of Chicago. Maria became a voluntary associate professor of physics there in 1946, but she received no salary because university anti-nepotism regulations forbade it. She also joined as senior physicist the research group at the Argonne National Laboratory near Chicago, where a nuclear reactor was being built. It was the first time she held a normal scientific post and she gladly accepted the opportunity to learn nuclear physics, a field in which she had had little experience. The University of Chicago was the first place where she "was not considered a nuisance, but greeted with open arms," she said. There she worked with Fermi, Urey, Franck, and Teller, especially on the calculations needed to solve the criticality problem for a liquid-metal breeder reactor. She programmed this calculation using the Monte Carlo method for ENIAC, the first electronic computer, which had recently been installed at Aberdeen. While carrying on her work at Argonne, she continued her voluntary role at the University of Chicago, which at the time hosted a group of very bright physical scientists, and where the exciting atmosphere in some ways must have been reminiscent of Göttingen in the early days.
Wartime research had produced a wealth of data about isotopes, atoms of the same element that have differing numbers of neutrons. Why some isotopes are more abundant than others became a lively issue among scientists. Unstable nuclei tend to decay radioactively, gradually changing from one element to another until they reach a stable form. Once they are stable, they do not change any more, so their numbers accumulate. Thus, the more stable the isotope, the more abundant it is in the universe. But why? Teller suggested that he and Maria find the answer, but he soon lost interest in the matter, rather focusing on the development of nuclear weapons and the origin of elements, while she was fascinated by a series of odd clues. Why, for example, does an isotope with 126 neutrons hold on to its neutrons more strongly than an isotope with 127 or 128 neutrons? Puzzle after puzzle, she noticed that certain nuclei were unaccountably abundant and therefore must be unusually stable and she found that they contained particular numbers of either protons or neutrons. The existence of ‘special numbers' in the nuclei of elements, which ultimately came to be referred to as 'magic numbers', had already been remarked in 1933 by the German physicist Walter Elsasser, but in 1948 much more was known about properties of nuclei than was available to Elsasser. The magic numbers not only stood up in the new data, but they clearly appeared in all kinds of nuclear processes.
Goeppert Mayer eventually discovered that in the especially abundant nuclei, either the number of protons or the number of neutrons equals one of the magic numbers, which proved to be 2, 8, 20, 28, 50, 82, 126. Although other scientists had discovered some of these magic numbers earlier, their existence was not considered important to nuclear structure. She was not aware of the earlier discoveries, and added new ones to the known list. As the numbers increased, the mystery deepened. It occupied Maria's mind day and night.
She began collecting more information on nuclei from nuclear experiments, and the data proved to be producing the very same numbers, pointing to a nuclear symmetry similar to that of an atom. She well knew that an analogous situation existed for atomic electrons surrounding the nucleus. Stability for atoms is chemical, since it is the loss, gain, or sharing of electrons that governs chemical reactions, during which the nuclei remain unaffected. Some nuclei had such tightly bound electrons that they do not combine easily with other chemical compounds by losing or gaining an electron. The periodic table shows that chemical properties recur in cycles, or periods, as the atomic number increases. The discrete energy states occupied by the electrons form a system, where each energy level is identified by a set of four quantum numbers, to which the restriction of Wolfgang Pauli's exclusion principle is to be added: only one electron can occupy a quantum state of a given set of quantum numbers. The outcome is that, as the atomic number rises, and electrons are added one by one, each additional electron occupies the next available level. Such energy steps occur in clusters of smaller steps, separated by unusually large steps. Such clusters were called shells. The chemical element in whose atom the outermost electron occupies the last level before a large step is said to "close the shell". The element of next higher atomic number (one more electron) starts the next shell. A closed shell represents a stable element: Because the addition or removal of an electron requires an unusually large amount of energy, chemical reaction is inhibited.
There had been speculations concerning the possibility of shell structure in nuclei dating from the discovery of the neutron in 1932. But the nucleus is very different from the atom as a whole. In the atom, the dominant central attraction occurs between the protons in the nucleus and the (relatively distant) orbital electrons. The nuclear forces between protons and between protons and neutrons within the nucleus, on the other hand, act at short range, and no single dominant centre of attraction exists. By 1936, it had become accepted by the majority of nuclear physicists that shell structure could not be valid in nuclei. At that time, Bohr had proposed his compound nucleus model of nuclear reactions, based on the assumption that all nucleons take part more or less equally in any nuclear process. The idea seemed natural, and was promoted by Bohr, who strongly opposed the individual-particle model. The experimental evidence of the time, notably the discovery of nuclear fission, pointed in the direction of a liquid drop model where the particles are closely coupled and did not favour a shell model. However, being an outsider to the postwar nuclear physics scene, Mayer was was looking at the problem afresh, and eventually demonstrated that two opposing nuclear models can coexist.
Even though she was only moderately familiar with nuclear physics, during her work on the Manhattan Project Goeppert Mayer had got an insight into the properties of fission products, such as decay energies and half-lives, so that after 1945 she began to look at the systematics of nuclear binding energies, and she discovered that they clearly indicated the presence of closed shells. Initially, she found two magic numbers, 50 and 82, soon followed by the other five, but no explanation for them.
Closed shells for nuclei with 2, 8, 20, 28, 50, 82 or 126 neutrons (or protons) clearly emerged from her work on nuclear binding energies. All these numbers are even, and in fact it had been known before that nuclei for which both N and Z are even are slightly more stable than those where one or both of these numbers are odd, because of the pairing effect, according to which nucleons like to couple to total spin 0. In an odd-A nucleus, the angular momentum is just that of the last odd particle. This rule, which Mayer investigated formulating a very simple model of the interactions between nucleons, involving an attraction of zero range, works well for most odd-A nuclei, and it was a major factor in the great success of her nuclear shell model.
The establishment of the particular values of the numbers indicating closed shells was the first major contribution of Mayer to the nuclear shell model. Even the skeptics were convinced by the overwhelming evidence, but there was no theory explaining why one got those particular values for the closed shell numbers. The lowest values 2, 8 and 20 could be obtained by putting nucleons into a reasonable single particle potential, like the harmonic oscillator, due to the other nucleons in which they move nearly independently. However, the higher numbers were a real challenge to explain. Several groups worked on this problem in 1948, trying to modify the potential so that the closed shells would appear for 28, 50, 82 and 126. The case of closed shells in nuclei has similarities to those of atoms, however, to account for the higher magic numbers, one had to take into account deviations from the oscillator potentials. Beyond the magic number 20, that can still be explained according to the atomic analogue, the system breaks down.
In this research, Goeppert Mayer was greatly encouraged by Enrico Fermi and had many discussions with him. Fermi was called "the oracle" within the Manhattan Project, because of his enormous knowledge and competence in all areas of physics and his legendary way to arrive at quantitive estimates for the solution of complicated physical problems. Moreover, he was the only physicist in Chicago who seemed interested in her work and with him she could debate any kind of topic in nuclear physics. One day, in 1949, they were in her office when Fermi was called to take a long-distance telephone call. As he reached the door, he turned and flung back a question: "Incidentally, is there any evidence of spin-orbit coupling?" Spin-Orbit coupling, the effect of the interaction of the electron's spin with the magnetic field created by the orbiting electron, plays an important role for the fine structure of atomic spectra, but had generally been assumed to be absent or in any case negligible in nuclei. Goeppert Mayer, who had lived with the nuclear data for a long time, recognized right away that this was the clue and answered: “Yes, of course and that will explain everything.” She knew she had hit on the final piece of the magic-number puzzle. Within ten minutes, before Fermi came back, she was able to begin the calculations that would prove what she knew was correct. She floated home, excited and finished her computations that very night: She discovered that when a strong spin-orbit coupling term is added to the nuclear potential, the sequence 28, 50 etc. for closed shells emerges naturally. The nucleus, too, could be depicted as having shells and described as "built up like an onion in layers", each magic number corresponding to a completed nuclear shell. Her solution was totally unexpected, because the spin of the electron has very little interaction with its orbital angular momentum, thus only determining the fine-structure, but not the shells themselves. Yet, inside the nucleus the spin-orbit coupling was showing to have a crucial effect.
The explanation of closed shells using the spin-orbit coupling also came, independently, to Hans Jensen, then a professor at Heidelberg University. Mayer and Jensen eventually became very good friends and later collaborated on a book, Elementary Theory of Nuclear Shell Structure, which was published in 1955. Eight years later, they shared half of the 1963 Nobel Prize for Physics "for their discoveries concerning nuclear shell structure." The other half was awarded to Eugene P. Wigner "for his contributions to the theory of the atomic nucleus and the elementary particles, particularly through the discovery and application of fundamental symmetry principles."
Maria Goeppert Mayer was at the time the only living woman in the world with a Nobel Prize in science, and she and Marie Curie were the only women to have won a Nobel in physics. Only shortly before, in 1959, she had finally been appointed to a position as a full professor of physics at the new campus of the University of California at San Diego.
Since then the nuclear shell model remains one of the cornerstones of modern nuclear physics. The success of Mayer’s independent-particle model for describing quantum systems in which the interactions are very strong stimulated deep work in the many-body problem. It later provided the intellectual background for the quark model.
Double Beta Decay
The theory of beta-decay developed in 1933 by Enrico Fermi by analogy with quantum electrodynamics, emphasizing a new mechanism based on particle creation and destruction processes, introduced a new type of interaction, weak interaction, to describe the process of beta-decay. Fermi's theory, the first modern quantum field theory, not only solved the long-standing problem of the mechanism of emission of electrons during the decay of radioactive nuclei, but it incorporated Wolfgang Pauli’s hypothesis on the existence of a neutral particle. This neutrino was associated to the electron during the decay process, and was capable of explaining the apparent failure of conservation laws as well as problems related to nuclear statistics. Soon after, in 1935, Goeppert Mayer was the first to propose the possibility of double beta decay, a process during which two neutrons in the nucleus are converted to protons, with transformation of the (A, Z) nucleus to the (A, Z+2) nucleus accompanied by the emission of two electrons and two neutrinos. Since the half-life for this process his huge (>100.000.000.000.000.000.000 years), it only plays a role in a few special nuclei that are otherwise stable. In 1937, Ettore Majorana theoretically demonstrated that all results of beta decay theory remain unchanged if the neutrino coincides with its anti-particle and another two years later Wendell H. Furry proposed that, if the neutrino is a Majorana particle, the double-beta decay can proceed without the emission of any neutrinos at all. The search for this process, now called neutrinoless double-beta decay, is one of the most interesting and challenging experiments in which hints at physics beyond the Standard Model of elementary particles might be discovered.
Bibliography
Bertch McGrayne S. (1993) Maria Goeppert Mayer. In Nobel Prize Women in Science. Birch Lane Press, New York, pp. 175-200
Ferry J. P. (2003) Maria Goeppert Mayer, Chelsea House Publishers, Philadelphia
Johnson K. E. (1986) Maria Goeppert Mayer: Atoms, Molecules and Nuclear Shells. Physics Today 39(9): 44-49
Goeppert Mayer M. (1963) The shell model, Nobel Lecture, December 12, 1963, available at http://www.nobelprize.org/nobel_prizes/physics/laureates/1963/mayer-lecture.pdf
Moszkowski, S. A. (2006) Maria Goeppert Mayer (1906-1972). In N. Byers and G. Williams (eds). Out of the Shadows. Contributions of Twentieth-Century Women to Physics. Cambridge University Press, Cambridge, pp. 202-212
Wasson, T. (ed) (1987) Mayer, Maria Goeppert. In Nobel Prize Winners, H. W. Wilson Company, New York, pp. 677-680
Perrin F. (1981) Joliot-Curie Irène. In Dictionary of Scientific Biography, Vol. 7. Gillispie C.C. (ed.), Charles Scribner's Sons, New York, pp. 157-159
Sachs R. G. (1979) Maria Goeppert Mayer. 1906-1972. Biographical Memoirs of the National Academy of Sciences : 311-328
Wigner E. P. (1972) Maria Goeppert Mayer. Physics Today 25 (5): 77-79