Prof. Dr. Otto Hahn > Research Profile
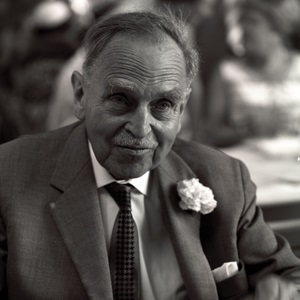
by Luisa Bonolis
Otto Hahn
Nobel Prize in Chemistry 1944
"for his discovery of the fission of heavy nuclei".
Becoming a Radiochemist
Otto Hahn was born on 8 March 1879 in Frankfurt-on-the-Main. At school he became attracted by chemistry and later he studied at the University of Marburg and Munich, receiving his doctor's degree in 1901 for a thesis in organic chemistry. After a few years as assistant, he set out for England in September 1904 to spend some time in the laboratory of Sir William Ramsay at University College, London. Ramsay, who was awarded the Nobel Prize in Chemistry that same year for his discovery of the noble gases, suggested that Hahn should work on radioactive materials, and gave him the assignment of separating radium from a relatively large quantity of barium salts, using Marie Curie's separation technique. Hahn's investigations of the radioactive decomposition of thorium resulted in the discovery of what at that time was believed to be a new radio element which he called radiothorium. In fact it was thorium-238, a still undiscovered isotope of the known element thorium, which he was able to concentrate in preparations several 100000 times as active as thorium itself.
Impressed by Hahn's successful achievement in the brand new field of radiochemistry, Ramsay recommended him to his friend Emil Fischer, director of the Chemical Institute at the University of Berlin, who had been awarded the 1902 Nobel Prize in Chemistry for his work on the constitution and configuration of carbohydrates. Fischer agreed to accept Hahn but suggested that he should spend six months with Ernest Rutherford at McGill University in Montreal, Canada, in order to improve his knowledge on radioactivity. Hahn arrived in Montreal in fall 1905, when Rutherford and Soddy had just concluded their collaboration, during which they had formulated the disintegration hypothesis and the general law of radioactive change. The science of radiochemistry was in its infancy: the identification of radioactive elements was based solely on rates of decay and intensities of radiation measured with fairly crude electroscopes. On Rutherford's suggestion, Hahn began to study the alpha-particle emission from the radiothorium and actinium preparations which he had brought with him from London. In measuring the alpha-particle range of radiothorium samples, Hahn discovered that a second alpha-emitting substance was present, which he called thorium C (now known to be polonium 214), and concluded that its half-life must be very short since all attempts at chemically separating it from radiothorium failed. He made similar measurements on his actinium sample, which pointed to the existence of another radioelement for which Hahn proposed the name radioactinium.
There can be no doubt that Hahn was profoundly influenced by his experiences, first with Ramsay in London and then with Rutherford in Montreal, from which he was able to learn the thinking processes and state-of-the-art methods of research in radioactivity. His early achievements also show that he had already become equipped with the great experimental skill that later led him to a preeminent position in the field.
Beginning to Collaborate with Lise Meitner
Hahn returned to Germany in the summer of 1906 to take up the appointment in the Chemical Institute at the University of Berlin that had been offered to him a year previously by Emil Fischer. In the space of a few months, he set up his laboratory in a large basement room and continued his investigations on thorium preparations, which soon revealed that his new substance, radiothorium, which he had identified solely on the basis of its alpha-radiation, could not be separated from thorium itself. Hahn was thus obliged to postulate the existence of yet another long-lived radioactive element, between thorium and radiothorium, to which he gave the name mesothorium. Because it was chemically inseparable from radium, which was difficult to obtain in Germany, and owing to the rising medical demand, it was marketed as “German radium.”
The discovery of new “elements” and the identification of “mother” or “daughter” relationships among them or with already known elements, provided only fragmentary evidence concerning the sequences of radiochemical transformations which were coming to light. It was also becoming apparent that various of these new so-called elements were chemically indistinguishable and inseparable, and that there were far too many of these to be accommodated within the periodic table.
In the spring of 1907 Hahn was appointed to the teaching staff in the Chemical Institute as Privatdozent, but he also had many good friends in the Institute of Physics, whose atmosphere he found quite stimulating. In September 1907, a young woman named Lise Meitner, who had studied with Ludwig Boltzmann in Vienna, came to Berlin with a Ph.D. in physics and some research experience in radioactivity. She had come to study theoretical physics with Max Planck, who was becoming the highest authority in Germany and was awarded the 1918 Nobel Prize in Physics. Meitner also wanted to pursue some studies in experimental radioactivity in the Chemical Institute. However, women were not allowed access to laboratories used by male students, so that Emil Fischer gave her special permission to work in the former carpenters' shop with Hahn. Since Hahn had an almost complete collection of known radioelements, they decided to examine the beta radiation and determine decay series for all these radioactive elements. Thus began their friendship, and a fruitful collaboration that lasted until Meitner was forced to leave Germany in 1938.
In October 1912 Hahn was given charge of a small independent Department of Radioactivity inside the Institute for Chemistry located in Berlin-Dahlem, which had only recently been established as part of the new Kaiser-Wilhelm Gesellschaft, together with the Institute for Physical Chemistry and Electrochemistry directed by Fritz Haber, who was awarded the Nobel Prize in Chemistry in 1918 for the synthesis of ammonia. When World War I broke out, Hahn was called up to serve with the gas-warfare corps, of which Fritz Haber served as scientific leader. During the war, whenever possible, he did some work in the radiochemical laboratory, where he had invited Meitner to join him. When she returned after serving as a audiologist in Austrian Army field hospitals, they began the experiments they had left in 1914.
They resumed their search for the mother substance of actinium, the unknown homologue of tantalum. They inspected in particular the leftover material resulting from the treatment of pitchblende with nitric acid and after careful work succeeded in understanding that it contained very small quantities of radium and other radioactive substances, but also virtually all the tantalum-like substances of the pitchblende. The suspicion that pitchblende residue was a useful raw material turned out to be correct. They succeeded in finding the unknown element and in showing it was the mother substance of actinium. Its formation was proved by the alpha-ray curves and by the measurements of actinium emanation, the steady increase of which was observed daily for a period of several months. An active deposit could be found in increasing amounts on negatively charged plates. The search for the previously unidentified radioactive element that decayed into actinium, took five years. A shorter-lived isotope of protactinium had been discovered in 1913 by Kasimir Fajans and Oswald H. Göhring, who named it brevium, afterward uranium X2, because it was a short-lived member of the uranium radioactive series. Meitner and Hahn proposed to name the long-lived isotope of element 91 proto-actinium , being a “parent of actinium”. Protoactinium (later contracted to protactinium) is actually one of the rarest and most expensive naturally occurring elements on Earth. For this discovery Hahn and Meitner were nominated many times for the Nobel Prize in Chemistry.
Like other researchers, Hahn and Meitner had recognized that certain groups of radioactive species appeared to have identical chemical properties. They were familiar with such facts as the inseparability of mesothorium and radium and of radiothorium and thorium. But theoretical speculations in general were not Hahn's style, even if he certainly was an outstanding experimentalist. The development of the concept of isotopes and the related radioactive displacement laws formulated in 1913 by Soddy - and independently by Kasimir Fajans - placed the radioelements in appropriate boxes of the periodic table, providing the clues for understanding the nature of the link between many of the numerous new radioactive elements. However, the relationships between decay products of uranium, as well as the link between uranium and protactinium still remained unknown.
By the early 1920s, almost all of the naturally occurring radioelements were known, and opportunities for basic research in radiochemistry were limited. Hahn began to turn his attention to possible applications of radioisotopes in various chemical fields and developed radiochemical methods which resulted in the founding of what became known as “applied radiochemistry”.
The Birth of the Nuclear Age
In 1919, by bombarding nitrogen with alpha particles from radium, Rutherford carried out the first artificial transmutation of a nucleus, creating “atoms of hydrogen” - hydrogen ions which he soon named protons - and, as was later proven by Patrick Blackett with his cloud chamber, oxygen nuclei, according to the reaction helium-4 + nitrogen-14 → oxygen-17 + hydrogen-1. In his famous Bakerian Lecture of 1920, Rutherford also argued that one nuclear electron might bind one proton, producing an “atom” of unit mass and zero charge: “Such an atom would have very novel properties. Its external field would be practically zero, except very close to the nucleus, and in consequence it should be able to move freely through matter.” He added that the existence of such atoms seemed “almost necessary to explain the building up to the nuclei of heavy elements.” Soon after, Rutherford moved to the Cavendish Laboratory, and encouraged attempts to reveal such a neutral particle. James Chadwick, since the beginning an enthusiastic believer in the existence of the neutron, announced the detection of the long-sought particle in February 1932. Ushering in the age of modern nuclear physics, the new nuclear constituent could at last readily explain the phenomenon of isotopy and led to further pioneering experiments and fundamentally new theoretical insights into the structure of the nucleus and opened the door to its potential use as an atomic projectile. Neutrons produced by bombarding beryllium with alpha particles emitted by radioactive materials began to be used to induce nuclear reactions. Their lack of electrical charge enabled them to strike nuclei without being repelled by their charge.
On January 15, 1934, a new startling discovery was communicated to the Paris Académie des Sciences. In bombarding an aluminium target with polonium alpha particles, Irène Curie and Frédéric Joliot discovered that their Geiger-Müller counter recorded the emission of positrons which persisted even after removing the polonium. They interpreted their surprising observation by assuming that a new radioactive isotope of phosphorous was created, which then decayed exponentially through the emissions of positrons. Similarly, when they bombarded other light elements with polonium alpha particles, radioactive isotopes were produced that also decayed exponentially with the emission of positrons, a process soon referred to as beta+ decay. They christened this phenomenon “artificial radioactivity.” A new and large field for further investigation was hereby opened. At the same time the experimental possibilities for carrying out nuclear physical investigations were fundamentally expanded.
The discovery of the neutron and the positron between 1932 and 1933, and now of artificial radioactivity, caused a real turmoil in the newborn field of nuclear physics. Immediately after Joliot and Curie's discovery of artificial radioactivity, Enrico Fermi in Rome made plans to repeat their experiments, but Fermi decided to see if neutrons, too, could induce artificial radioactivity. Being uncharged, Fermi thought they would be able to penetrate even into the nuclei of highly packed atoms and to transform them in the same way that happens in natural radioactivity. He obtained positive results with aluminium and fluorine, and in April 1934, working with his collaborators, he found that his neutron source produced neutron-induced artificial radioactivity in thirteen additional elements. The results were processes with the expulsion of either alpha particles, protons, or gamma radiation. According to the displacement laws of Soddy and Fajans, the alpha-decay should lead to an isotope whose place in the periodic table is two positions below that of the parent, that is of the bombarded element, while the daughter of a beta emitter takes the place of the subsequent element.
Fermi's subsequent achievement in the following October was his discovery of the increased efficacy of neutrons in producing nuclear reactions when slowed down in their passage through a hydrogenous substance like paraffin. As Fermi immediately understood, slow neutrons were more likely to interact with nuclei than fast neutrons. They could easily slip into an atom and be absorbed by the nucleus, making an isotope heavier by one atomic mass unit. The newly constructed nucleus would usually emit a beta particle (a negative electron) and thereby transmute to a more stable isotope, with an atomic number one unit higher.
Since spring 1934, proceeding systematically according to increasing atomic number, Fermi and his collaborators at length also bombarded thorium and uranium with neutrons, and as with the other elements, neutron capture resulted in the production of beta-emitting radioisotopes, which could not be easily interpreted. Since emission of a beta particle leaves the target nucleus with one more unit of positive charge, and radiochemical evidence seemed to exclude the possibility that they might be isotopes of radium or actinium or other lighter elements, Fermi's attitude was to try to find an interpretation in terms of what he had learned during the last few months without introducing “ad hoc” assumptions, such as new nuclear processes. Therefore, the simplest way was to interpret their results as due to a transuranic element Z=93 generated in the beta decay of a radioactive nuclide produced by radiative capture in uranium-238 (uranium-235 was discovered the following year and uranium-234 makes up only a very small percentage of the raw uranium). They assumed this to be an element with chemical properties similar to those of the rare metal rhenium. Soon after, they studied another beta-emitter, suggested to be an isotope of element 94, which they assumed to be homologous to osmium, a transition metal in the platinum family. The possible production of two transuranic elements was suggested with considerable caution in published articles, so that Fermi was deeply disturbed by the publicity given by the Italian press, celebrating the discovery as a great triumph of fascist science.
In the scientific community, controversy arose from this proposal. In 1934, Ida Noddack , a chemical expert on rare earths and, together with her husband, co-discoverer of the element rhenium, noted that Fermi's chemical tests were not conclusive in eliminating all elements slightly lighter than uranium. In questioning Fermi's tentative conclusion, she suggested that, since the effects of neutron bombardment were still poorly understood, it was conceivable that a different reaction might be occurring, namely breakup of the nucleus into “several large fragments, which could be indeed isotopes of known elements, but not neighbours of the irradiated elements.” Chemical analyses were not done by Fermi's group and, though she was a chemist, Noddack did not reproduce Fermi's experiments to look for such evidence. Her suggestion probably appeared as a speculation aiming more to point out a lack of rigour in the argument for the formation of element 93. Because her idea went against the conventional wisdom that no particles heavier than alpha particles could be emitted, it was not taken seriously at the time. Moreover, she and her husband insisted on their detection of element 43, which they called “masurium”, and which nobody was able to reproduce, a circumstance that considerably undermined their scientific credibility. According to what was later recalled by Emilio Segrè, a member of Fermi group in Rome: "The reason for our blindness, shared by Hahn and Meitner, the Joliot-Curies, and everybody else working on the subject, is not clear to me even today."
The “Uranium Puzzle”
After her initial collaboration with Hahn, Lise Meitner developed her own independent research into beta-spectra, and both had continued to co-operate to a limited extent on studies of beta-radiation from radium and radioactinium. She became Dozent in 1918 and extra-ordinary professor in 1926, the first woman university physics professor in Germany. After 1934, they were working separately, Hahn the head of the Kaiser Wilhelm Institute for Chemistry and Meitner head of that institute's physics department. But Meitner was well acquainted with Italian physicists and regularly received preprints of their articles, so that she proposed to Hahn that they resume their old collaboration, after a lapse of nearly fifteen years.
In fact, another objection to Fermi's interpretation came from Aristid von Grosse, a former colleague of Hahn's. According to Fermi's assumptions, element 93 should be similar to rhenium, so that von Grosse had argued that some of the active species found by Fermi might be isotopes of the very rare element 91, protactinium, because he had found this element - which he had just isolated - to be carried by rhenium sulphide. In order to work with the very small invisible amounts of radioactive elements produced by irradiation with neutrons - detectable only by their radioactivity - the radiochemical carrier technique was in fact used, according to which a small quantity of a homologous element was added to the solution containing the irradiated sample in the form of a salt, base or acid conveniently chosen. The solution thus contained large amounts of the original nuclides, plus the products of all nuclear reactions initiated by the incident radiation. Then a characteristic operation for that carrier element, such as a precipitate reaction was performed. If the radioactivity accompanies the carrier, the radioactive element has similar chemical properties. This was a typical chemical process used to establish the chemical identity of the product of a nuclear reaction. As discoverers of protactinium, Hahn and Meitner were familiar with its chemical properties and thus especially intrigued by Fermi's experiments on uranium; in particular they hoped to use uranium-Z as tracer for protactinium. Hahn was at the time the world's most experienced radiochemist and, as an authority in the radiochemistry of the heavier metals, he was quite interested in investigating the matter further. Chadwick's discovery of the neutron, the Curie and Joliot's discovery of artificial radioactivity, and Fermi's use of neutron bombardment to produce additional radioactive materials, including some thought to be new elements beyond uranium in the periodic table, were resurrecting radiochemistry, and transforming it into nuclear chemistry, a new research field which Hahn and Meitner were entering with outstanding experience and skill.
As a chemist, Hahn was delighted to have lots of news "transuranium" elements to study, it was like the old days, when "new elements fell like apples when you shook the tree." Hahn and Meitner thus entered the game and began their own experiments irradiating uranium with neutrons. They definitely showed that Fermi's isotope was not an isotope of protactinium, and quickly confirmed by chemical investigations that the elements produced when bombarding uranium-238 with neutrons were not any of the elements from uranium (Z=92) to mercury (Z=80). They concluded, like Fermi, that the elements produced had to be transuranic elements of atomic number 93 or higher, adding to a growing consensus among scientists. Detecting and identifying a few thousand atoms required both standard radiochemical techniques and consummated skill, which Hahn in particular had gained step by step after starting with his work with Rutherford at Montreal. But with such minuscule amounts, which could only be detected by the ionizing effects of their radiation, it was extremely difficult by the methods of chemical analysis to gain a clear understanding of their chemical properties, even for Hahn and his collaborators, who were masters of such methods. At the same time, the confident expectation that the products would be found among the restricted set of elements whose atomic numbers were within a few units of the atomic number of the bombarded substance represented a main guiding principle for tackling the puzzles of transmutation.
The work of the Berlin team was interdisciplinary, requiring nuclear physics for the reaction processes and chemistry and radiochemistry for analysing the many radioactive products contained in their precipitate, so that in 1935 Fritz Strassmann, a young analytical and physical chemist whose scientific talents in separation chemistry complemented those of both Meitner and Hahn, joined the work. Hahn, Meitner, and Strassmann tried to unravel the multiple series of decays that occurred in the sample of irradiated uranium and in 1937 they published a paper showing that three families of transuranic elements were necessary for interpreting the intriguing uranium puzzle. For some time they were convinced that they had produced a series of transuranic elements with atomic numbers up to 95, with possible extensions even to elements 96 and 97.
However, irradiated uranium produced complex products and nobody knew just what the chemistry of transuranium elements should look like. At that time, the actinide series was not yet known in the periodic systems, and thus all elements after actinium were classified incorrectly. The most straightforward guess was that the periodic table could simply be extended downward: if uranium was regarded as lying beneath the similar metallic element tungsten, then the first element beyond uranium, number 93, should lie beneath the element next to tungsten, namely rhenium and so on, with elements homologous to osmium, iridium, etc. In these attempts to make transuranium elements, two premises were always made which seemed to be firmly established by theory and experiment. First, in nuclear transformations, spontaneous and induced, atomic numbers are changed very little leading only to reaction products in the neighbourhood of the transformed element. Second, it was not yet known whether the periodic system would show another transition group analogous to the rare-earth group that begins with lanthanum, and if so where it would start. So the exact position of transuranic elements in the periodic system - and hence their exact chemical behaviour - was not yet known. Furthermore, since radioactive alpha decay was understood as a quantum mechanical tunnelling process through a Coulomb barrier, the emission of larger nuclei seemed extremely unlikely. How could a substantial fragment escape transforming a uranium nucleus into a substantially lighter nucleus? The problem was thus full of pitfalls. However, by 1938 the nuclear physics community had little doubt about the existence of the transuranium elements, which were treated as an established fact in textbooks, articles, public lectures.
In the meantime, Irène Curie, who had soon tackled the problem of the artificial radioactivity induced by neutrons, and was now working with the physicist Pavel Savitch, also began exposing thorium and uranium to neutron sources. Between 1937 and 1938 they stated that they had obtained, by irradiation of uranium with neutrons, a so-called 3.5 hour substance whose chemical properties could not readily be determined, but it appeared to be those of lanthanum. After performing new experiments, they decided, with some hesitation, to include the substance in the transuranium series, with atomic number 93, but the possibilities put forward appeared difficult to understand and unsatisfactory. In particular, it was not clear how it would fit into Hahn, Meitner and Strassmann's scheme of decay chains. The Curie-Savitch paper was thus a challenge to the Berlin group. However, when the Nazi regime annexed Austria in early 1938, Meitner's situation in Germany had been changed. Even though she was “over 50 per cent non-aryan,” Meitner, as an Austrian citizen, had been protected from the Nazi Civil Service Laws. With the Anschluss of 12 March 1938 those laws suddenly applied to her and she could expect to be dismissed from her post and to suffer other penalties. She was refused an exit visa and a plan was then immediately prepared to get her out of Germany secretly. She left Berlin-Dahlem on 17 July 1938 and went to Sweden, invited by Manne Siegbahn, the 1924 Nobel Prize laureate in Physics. The last joint paper by Meitner, Hahn and Strassmann had been sent to the editor of Die Naturwissenschaften only a week before she left Germany.
The Discovery of Nuclear Fission
Curie and Savitch's last paper on the mysterious "3.5 substance", first thought to be thorium then actinium and finally a transuranic with lanthanum-like properties, reached Hahn and Strassmann in October 1938. In contrast to earlier papers from Paris, it contained detailed decay curves, which spoke for themselves. They immediately began to test their results. After careful experiments they concluded that, in addition to the transuranic elements they had already found in previous experiments, there were - produced by two successive alpha-emissions - three artificial, beta-active radium isotopes with different half-life times, which in their turn changed into artificial beta-active actinium isotopes. When identifying these processes, radium had been precipitated using barium as the carrier element, because it is homologue of radium, and hence radium remains closely bound to the carrier in precipitation. According to the chemical properties, only barium and radium could thus be considered, and those separated by means of a barium precipitate could only be isotopes of radium (Z = 88), since barium itself (Z=56) was out of the question, according to the conservative viewpoint of the time. It was in any case difficult to explain how could one pass from uranium, number 92 in the periodic table, to radium, number 88, especially as no alpha particles were observed.
On 8 November they reported their preliminary findings and the starting result of the appearance of radium isotopes and their actinium decay elements, and a few days later Hahn secretly met Meitner in Copenhagen. She urged him to double-check his radium data. Thanks to great weight they gave to her opinion and judgment, the two chemists in Berlin immediately undertook the necessary control experiments. On 19 December 1938, Hahn wrote to Meitner saying that the attempts to separate their supposed artificial “radium isotopes” from the barium carrier through the elaborate procedure of fractional crystallizations and fractional precipitations had been unsuccessful: "There is something about the radium isotopes that's so curious that we wanted to first tell it only to you." Accurate tests showed that the fractionation procedure was not at fault: “We more and more come to the terrible conclusion that our radium isotopes do not behave like radium but like barium.” But how could barium (Z=56) arise from the irradiation of uranium (Z=92)? Two days later, Meitner replied, "Dear Otto, your radium results are very puzzling […] However, we have experienced many surprises in nuclear physics, so that one cannot say without further consideration that it is impossible. In a further letter sent on 21 December, Hahn said that as chemists they had no doubt of the accuracy of their work, and had been forced to conclude that the substance previously thought to be radium was in fact an isotope of the much lighter element barium and likewise that the supposed actinium isotopes were isotopes of lanthanum: “We cannot completely hush up our results, even if they are perhaps physically absurd.” He asked her to find some sort of “fantastic explanation” for the surprising result, and added: “If you can propose something that you can publish, then it would still be a sort of cooperation of us three.”
During an excursion through the woods, Meitner discussed the letter with her nephew Otto R. Frisch, who was visiting her for Christmas coming from Copenhagen, where he worked in Niels Bohr's Institute. Meitner was convinced that Hahn was too good as a chemist and that the result was correct. But how can one get a nucleus of barium from one of uranium?They worked out what this conclusion would mean in terms of nuclear physics and provided the first theoretical interpretation of what they understood to be a novel type of nuclear process, completely unforeseen, but explainable on the basis of the already existing liquid-drop model of the nucleus formulated by George Gamow and Bohr. In adding sufficient energy to the uranium nucleus, the neutron would, in some cases, cause it to deform into an elongated shape. Mutual repulsion of the positive charges at each end of the elongation would overcome the surface tension and break the drop at its narrow neck. They made a rough calculation of the mass loss, which agreed with a similarly rough value for the kinetic energy of the fragments. The energy released was about 200 million electron volts, whereas the most energetic chemical reactions involve but a few electron volts, and ordinary radioactive processes involve only several MeV.
In the meantime, on December 22, Hahn and Strassmann sent a communication to Die Naturwissenschaften, discussing their recent experiments which they actually had published “with reluctance because of the extraordinary results.” In fact, “as chemists”, they had been led to conclude that the their group of substances in their previous scheme had to be renamed: “[...] instead of Ra, Ac, Th [radium, actinium and thorium], write the symbols Ba, La, Ce [barium, lanthanum and cerium].” The uranium nucleus appeared to have been split into fragments consisting of lighter elements, lying very far from uranium in the periodic table. As “nuclear chemists”, somewhat closely related to physics - they added - “we cannot yet make up our mind to undertake this leap, which contradicts all previous experience in nuclear physics. Perhaps it is still possible that a number of strange chance occurrences have counterfeited our results [added emphasis].” Their short article, which appeared in the 6 January 1939 issue of Die Naturwissenschaften, was now making public the existence of a completely unsuspected nuclear process and stirred an incredible turmoil in the world of physicists.
On January 1, in receiving a carbon copy of Hahn's proofs, Meitner wrote from Stockholm: "I am now almost certain that the two of you really do have a splitting to Ba and I find that to be a truly beautiful result, for which I most heartily congratulate you and Strassmann […]. Both of you now have a beautiful, wide field of work ahead of you. And believe me, even though I stand here with very empty hands, I am nevertheless happy for these wondrous findings."
Ushering into the Nuclear Age
When Frisch returned to Copenhagen and began explaining the splitting of the uranium nucleus to Bohr, the latter immediately exclaimed, “Oh, what idiots we have been that we haven't seen that before!” In Paris, Irène Curie commented “What fools we were!” Frisch learned that the process of cell division was called fission by biologists. Soon after Hahn and Strassmann's first publication on the production of barium from uranium, there appeared an article titled “A new type of nuclear reaction,” signed by Lise Meitner and Otto R. Frisch. In this article the term “fission process” appeared for the first time and the possibility of a breakdown of heavy atomic nuclei into two lighter ones, with total charges equal to that of the original nucleus, was explained. On January 13, 1939, Frisch also successfully performed a simple experiment, in which he was able to observe the large ionization pulses caused by the fission fragments. The Berlin team's chemical evidence was now supported by physical data. Now it was clear what kind of physical phenomenon one should look for.
During searches for energetic alpha particles emitted from uranium or thorium bombarded with neutrons carried out in several laboratories, aluminium foils had been put in front of the uranium and thorium samples surrounded by paraffin in order to eliminate the background of natural alpha particles. It was precisely this foil that prevented physicists from observing the large characteristic ionization pulses due to fission fragments. In retrospect, however, it is impossible to say whether they would have correctly interpreted the phenomenon had they observed it.
By the end of 1939 over a hundred articles relating to nuclear fission had been published all over the world, inaugurating the advent of the nuclear age, a real watershed in human history.
On November 16, 1945 the Royal Swedish Academy of Sciences announced that Hahn had been awarded the 1944 Nobel Prize in Chemistry “for his discovery of the fission of heavy atomic nuclei”. However, Hahn was still detained at the English estate known as Farm Hall by the Allies, and his whereabouts were a secret. It was thus impossible for the Nobel committee to send him a congratulatory telegram and Hahn learned about his award through the Daily Telegraph newspaper. It was only the following December 1946 that King Gustav V of Sweden finally presented him with his Nobel Prize medal and diploma.
Hahn's Nobel lecture traced the course of scientific research tat led from the natural transmutations of uranium discovered by Henri Becquerel to nuclear fission. He concluded reading part of Frédéric Joliot's 1935 Nobel lecture, in which the French physicist had warned against the potential hazards of atomic energy, and which by that time had already become "a threatening reality,” with the nuclear bombing of the Japanese towns of Hiroshima and Nagasaki. The event had actually caused Hahn a great shock and a period of depression. He stressed that the scientists of the world should strive toward the peaceful use of nuclear energy.
In 1946 Hahn became president of the Kaiser Wilhelm Society, which was renamed the Max Planck Society, and played a major role in reestablishing not only the society's research institutes but German science as a whole. In 1947, Meitner refused Strassmann's invitation to return to Germany to direct her old institute, which had been renamed the Max Planck Institute for Chemistry and moved to Mainz. In 1959, on Hahn's eightieth birthday, it was announced that the Institute of Nuclear Research in Berlin would be renamed the Hahn-Meitner Institute and that the Max Planck Institute for Chemistry in Mainz would become the Otto Hahn Institute.
In 1966 the United States Atomic Energy Commission awarded its Enrico Fermi Award to the entire old Berlin team: Hahn, Meitner, and Strassmann. It was the first time that non-Americans had received the prize and the first time that a woman had won it. The element 109, that at the time of its discovery in 1982 was the heaviest known element in the universe, in 1997 was officially named meitnerium in honour of Lise Meitner.
Bibliography
Badash L. (1981) Hahn, Otto. In Dictionary of Scientific Biography, Vol. 6. Gillispie C.C. (ed.), Charles Scribner's Sons, New York, pp. 14-17
Hahn O. (1958) The Discovery of Fission. Scientific American 198: 76-84
Hahn O. (1966) A Scientific Autobiography. Charles Scribner's Sons, New York
Herrmann G. (1989) Discovery and confirmation of fission. Nuclear Physics A502: 141c-158c
McBryde W.A.E. (1993) Otto Hahn. in K.J. Laylin (ed.), Nobel Laureates in Chemistry. 1901-1992.
American Chemical Society and the Chemical Heritage Foundation, pp. 272-281
Sime R.L. (2008) Hahn, Otto. Gale Virtual Reference Library. http://go.galegroup.com/ps/i.do?id=GALE%7CCX2830905718&v=2.1&u=mpiwg&it=r&p=GVRL&sw=w
Sime R.L. (1996) Lise Meitner: A Life in Physics. University of California Press, Los Angeles
Spence R. (1970) Otto Hahn. 1879-1968. Biographical Memoirs of Fellows of the Royal Society 16: 279-313