Prof. Dr. Antony Hewish > Research Profile
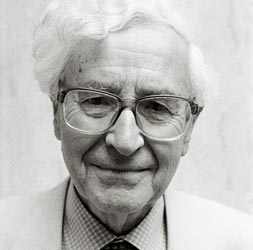
by Luisa Bonolis
Anthony Hewish
Nobel Prize for Physics 1974 tgether with Martin Ryle "for their pioneering research in radio astrophysics: Ryle for his observations and inventions, in particular of the aperture synthesis technique, and Hewish for his decisive role in the discovery of pulsars".
Twinkle, twinkle little star: becoming a radio astronomer
Anthony Hewish was born in Fowey, Cornwall, in 1924. After attending King's College in Taunton, he entered Cambridge University in 1942, but the following year he left college for wartime work. He first worked on radio receivers, but after a few months he was transferred to the Telecommunications Research Establishment in Malvern (TRE), which was the top-secret centre for the development of airborne radar devices. The leader of the Malvern team was Martin Ryle, an Oxford scientist who was at that time working on the design of antennas for airborne radar equipment. Hewish’s main job was to visit the Bomber Command airfield where this equipment was first installed and to instruct the RAF technicians how to use it. He found the teaching experience fulfilling and during three years of war service he began to develop a real understanding of physics.
After the war, Hewish returned to Cambridge and after he graduated in 1948 he was offered a research studentship at Cavendish Laboratory. It was an exciting era, when radio astronomy began to develop from a specialist pursuit of physicists and electrical engineers into a key area of contemporary astronomy. After the Second World War, a number of university groups began the investigation of the nature of the cosmic radio emission, which had been discovered by Karl Jansky in 1932. He detected cosmic radio noise from the centre of the Milky Way Galaxy while investigating radio disturbances that interfered with transoceanic telephone service. The American amateur radio operator Grote Reber later built the first radio telescope and found that the radio radiation came from all along the plane of the Milky Way and from the Sun. For the first time, astronomers could observe objects in a new region of the electromagnetic spectrum outside that of visible light. The principal radio groups involved in post-war research activity were those at Cambridge, Manchester, and Sydney. The Cambridge efforts were led by Martin Ryle who had just discovered four radio “stars”. These were mysterious objects having no apparent connection with visible stars. Jack Ratcliffe, head of radiophysics at the Cavendish Laboratory, had given an excellent course on electromagnetic theory during Hewish's final undergraduate year and had directed his activities at TRE. Ratcliffe told him that Ryle was looking for a new research student to work on the strange radio stars. Hewish was already familiar with the technology required and had a great respect for Ryle's scientific brilliance and drive. He thus joined Ryle's radio astronomy research group at the glorious Cavendish Laboratory at Cambridge.
Hewish's first task as a new student was sawing a large pile of brass tubing into pieces and then soldering them onto copper wires to make a dipole array. Ryle's speciality was the use of two such arrays separated by a considerable distance and connected together to form an interferometer. Radio interferometry was used at that time to perform the first high-resolution radio astronomy observations. In this technique, the data from each antenna are combined (or “interfered”) and joined to the same receiver. Coincident signals reinforce one another, while conflicting signals cancel each other out in a manner analogous to the way fringes are produced in the optical interferometer. The principle of the radio interferometer's operation is the same as for an optical interferometer, but, because radio waves are much longer than light waves, the scale of the instrument is generally correspondingly greater. The effect is to achieve the collecting power of a single large instrument encompassing the individual collecting sub-components. Today, the technique has evolved into powerful radio interferometers such as the Very Large Array located on the Plains of San Augustin in central New Mexico, consisting of 27 parabolic antennas, each measuring 25 metres in diameter, with a total collecting area equivalent to a single 130-metre antenna.
Hewish was lucky to participate in these pioneering efforts and to share in the team spirit, which became a notable characteristic of Ryle's group for many years. Following a suggestion from Ryle, he started by setting up a simple antenna to measure the polarisation of intense bursts of solar radiation emitted when there were large sunspots present, a radiation which jammed the radars in 1942. But he soon got bored with waiting for solar activity to occur and got involved with a much more exciting problem. The first few radio stars had been observed to vary in intensity - they sometimes scintillated on a timescale of seconds to minutes, twinkling rather like ordinary stars. Was this an intrinsic effect due to physical changes within the sources or was it caused by propagation through the atmosphere?
Ryle asked Hewish to study this as his first research problem and he found that the twinkling was pronounced only during a few hours around midnight. Following up this phenomenon, which had not been noticed before, Hewish next found that the occurrence of twinkling was associated with disturbed conditions high up in the ionosphere - the ionised zone of the upper atmosphere, which reflects radio waves and is important for long-distance communications. Once Ryle realised that the twinkling was unconnected with the radio stars themselves, he was no longer very interested and Hewish saw the chance to develop his own field of research, which happened to relate more closely to work being done by Ratcliffe's team. Edward Appleton, who had been awarded the Nobel Prize in 1947 for his research on the ionosphere, had pioneered radiophysics at the Cavendish, and Ratcliffe was continuing this work. Hewish's interest was attracted by the fact that radio waves from radio stars must traverse the whole thickness of the ionosphere and could therefore give information unobtainable using the standard methods involving waves transmitted from the ground and reflected from the underside of the ionosphere. Moreover the transmitters were provided free by nature. But first he had to work out the necessary theory. Inspired by Ratcliffe's superb lectures on Fourier analysis, it suddenly occurred to him that there was an exact analogy between a well-known theorem and his problem concerning radio waves traversing the ionosphere. He then realised how the observations of twinkling could be related to the size and height of turbulent clouds in the ionosphere in a quantitative manner using Fourier methods. He developed the theory of diffraction by phase-modulating screens and set up radio interferometers. Using a pair of simple radio telescopes separated by about 1 km and timing the variations of intensity at each site, he was able to make pioneering measurements of the height and physical scale of plasma clouds in the ionosphere and also able to estimate wind speeds in this region. Hewish has recalled how exciting it was: “cycling home one moonlit night and savouring the thrill of realising that I was the only person in the whole world to know how the wind was blowing at heights of three hundred kilometres.”
Ratcliffe was highly impressed by Hewish’s theory and encouraged him to write two papers, which were published in the Proceedings of the Royal Society of London. So this research became the main topic for his doctoral dissertation and resulted in further publications.
After earning his Ph.D. for his radio studies of the upper atmosphere in 1952, ionospheric research could have continued as a major interest, but Hewish was diverted by another development. By the early 1950s, it had been found that most of the so-called radio stars were actually a new kind of galaxy, rare, powerful, and at huge distances. Others were
the remains of exploding stars called supernovae, which left behind radio-emitting clouds of hot gas. At that time, Ryle was searching for ways of making more powerful radio telescopes. A new type of radio telescope was constructed which was designed to improve the accuracy of the positions of the weaker sources and thus to enable a greater number of identifications to be made, and also to make possible the observation of a considerably larger number of sources than in previous surveys. The instrument made use of four aerials situated at the corners of a rectangle and the resulting interference patterns in the north-south and east-west planes enabled the coordinates of radio stars to be determined with great precision. In addition, the system could be arranged to detect sources of large angular diameter and to investigate the general background radiation. The first multi-element astronomical radio interferometer was used for accurate location of weak radio sources. With improved equipment, Ryle observed the most distant known galaxies of the universe, guiding the Cambridge radio astronomy group in the production of radio source catalogues. The Third Cambridge Catalogue (1959) contained some sources, most notably 3C 273, that were identified with faint stars. In 1963, the American astronomer Maarten Schmidt observed 3C 273with an optical telescope and found that it was not a star in the Milky Way Galaxy but a very distant object nearly two billion light-years from Earth, receding with a velocity greater than that of any other known celestial object. Objects like 3C 273 were then called quasi stellar-radio sources, or quasars. They are the most energetic and distant members of a class of objects called active galactic nuclei. There is now a scientific consensus that a quasar is a compact region in the centre of a massive galaxy, which surrounds its central supermassive black hole. The energy emitted by a quasar is believed to derive from mass falling onto the accretion disc around a black hole.
Hewish did a great deal of antenna design and testing for Ryle's first radio telescopes along with his own research. In the early 1950's, colleagues in their group, and also Russian radio astronomers, had found that radio waves from the Crab Nebula were affected by the solar corona for a few days each June when this source was located at a small angle from the Sun. Hewish realised that the solar corona could affect radio waves passing through it similarly to the ionosphere. He set up special observations that confirmed that the solar atmosphere was responsible and applied his theory to learn about clouds in this hot gas surrounding the sun. He found that the source was being blurred, so that instead of twinkling it was refracted into a wide disc, just like when a distant streetlight is seen through the dimpled glass of a bathroom window. Until then it had only been possible for optical astronomers to see the corona during rare total eclipses and very little was known about the sun's atmosphere, so Hewish thought it would be exciting to see how far out into space it extended. So he stopped working on the ionosphere and began to build his own radio antennas at sites up to ten kilometres from the observatory. This was essential, as he had to use the interferometers to measure the small refraction at great distances from the sun and the observatory site was too small to contain both antennas. It was incredibly exciting organising his own small team and running an independent research programme. Eventually, Hewish was able to study the solar atmosphere out to one half the distance to the Earth.
In 1964 came another breakthrough, when Ryle's group noticed that some radio galaxies showed scintillation during daytime, which could not be related to the ionosphere. He wondered if the solar atmosphere might be causing this twinkling to occur. A few of these sources coincided with quasars. Quasars had shown unexpected variations of intensity, and Hewish realised that these sources might, indeed, have a small enough angular size for the solar atmosphere to cause the scintillation. They immediately checked this by special observations and found that the rate of variation of intensity fitted his theory rather well, provided that the speed of the solar wind was roughly the same near the sun as they are farther out. The speed of distant solar winds had been detected by spacecrafts between the end of the 1950s and the beginning of the 1960s. Spacecrafts, however, were limited to observations in the plane of the Earth's orbit due to constraints set by energy requirements at launch. By repeating the techniques that he had used earlier for the ionosphere, Hewish was able to overcome this limitation and measure the wind emitted from the Sun's polar regions. Since the solar wind was so fast, it was necessary to set up radio telescopes at sites one hundred kilometres apart, and to use larger antennas. By choosing suitably located radio galaxies so that the line of sight passed over the solar pole, they found an enhanced wind speed in this region. Thirty years were to elapse before the space probe Ulysses, a joint venture of NASA and the European Space Agency, could orbit the Sun and study it at all latitudes, confirming his finding.
Another application of interplanetary scintillation that Hewish wanted to exploit was its use in finding more quasars, as these turned out to be sources of prodigious energy. In the early 1960s, radio telescopes were unable to obtain sharp enough images to distinguish between quasars and normal radio galaxies, whereas scintillation gave a direct indication of their tiny angular size. A highly sensitive radio telescope was needed to detect a large number of faint radio galaxies. His experience with setting up simple but effective antennas for studying the solar wind showed how a large array of similar structures could be combined to produce the necessary sensitivity. So in 1965 Hewish drew up plans for a radio telescope with which he intended to carry out a large-scale survey of more than 1000 radio galaxies using interplanetary scintillation to provide high angular resolution. To achieve the required sensitivity it was necessary to cover an area of 18 000 square meters. The final design was an array containing 2048 dipole antennas arranged in 16 rows of 128 elements. Each row was 470 m long and the north-south extent of the array was 45 m. Later that year he was joined by a new graduate student, Jocelyn Bell, who became responsible for the network of cables connecting the dipoles. The entire array was connected by 120 miles of wire and cable.
“Little Green Men”... or a new kind of astrophysical object?
The commissioning of the 4.5-acre array proceeded through the summer of 1967. Hewish suggested that Bell create sky charts for each strip of the sky each day, noting all the scintillating sources. If the scintillating sources were present on successive weeks at the same astronomical coordinates, they were likely to be real sources, whereas if the scintillation were simply the result of interference with other sources, they would not recur at the same astronomical coordinates. This was a very demanding task requiring great persistence, patience, and attention to detail on Bell’s part since she had to keep up with the high rate at which the charts were being produced by the telescope. The radio telescope was completed and tested by July 1967, and they immediately began a survey of the sky, making repeated observations so that interplanetary scintillation could be observed over a wide range of angular distance from the Sun for any radio galaxy. They surveyed the entire range of accessible sky at intervals of 1 week. To maintain a continuous assessment of the survey, they arranged to plot the positions of scintillating radio sources on a sky chart, as each record was analysed, and to add points as the observations were repeated at weekly intervals. In this way genuine sources could be distinguished from electrical interference since the latter would be unlikely to recur with the same celestial coordinates. This is a feature of all radio astronomy. Radio telescopes are very sensitive instruments, and it takes little radio interference from nearby on earth to swamp the cosmic signals.
One day around the middle of August 1967, Bell became aware that on occasions there was a bit of “scruff” on the records which did not look exactly like a scintillating source, and yet did not look exactly like man-made interference either. Furthermore, in examining previous recordings, she realised that this scruff had been seen before on the same part of the records, from the same patch of sky. The source was transiting during the night, a time when interplanetary scintillation should be at a minimum.
They first thought that the signals might be electrical interference. However, by the end of September, routing survey records showed that the source sometimes appeared on the sky map in the same position, but occasionally it was not present. More detailed examination revealed that it was emitting pulses of radio waves of a very stable frequency. Another odd fact was that it scintillated too strongly. Whatever it was they decided that it deserved closer inspection, and that this would involve making faster chart recordings as it transited. Towards the end of October, Bell started going out to the observatory each day to make the fast recordings. They were useless. For weeks she recorded nothing but receiver noise. The “source” was apparently gone. Then one day she skipped the observations to go to a lecture, and next day she saw the scruff had been there. A few days after that, at the end of November 1967, she got it on the fast recordings and with this improved time-resolution the pulses were detected separately for the first time. She immediately saw that the signal was a succession of short pulses repeating at regular intervals. Hewish's first reaction was that they must be man-made. The pulses appeared again the next day and Hewish checked the recording establishing that this signal, whatever it was, kept accurately to sidereal time. But a sequence of pulses with repetition period 1.33 seconds apart seemed suspiciously man-made. Besides it was far too fast a pulsation rate for anything as large as a star. It could not be anything earth-bound because it kept sidereal time. Hewish could not believe that any natural source would radiate in this fashion and he immediately consulted astronomical colleagues at other observatories to inquire whether they had any equipment in operation that might possibly generate electrical interference at a fixed sidereal time near 19 h 19 m. They also considered and eliminated radar reflected off the moon into their telescope, satellites in peculiar orbits, and other anomalous effects caused by a large, corrugated metal building just to the south of the telescope area.
They spent a whole month trying to find out what was wrong, so unexpected was the signal, whose period was found to be stable to better than 1 part in 106. They speculated that the signal might possibly be from a distant civilisation, and nicknamed it “Little Green Men”. Then, John Pilkington in their group measured the bandwidth of the signal from which he estimated that the source was well outside the solar system but inside the galaxy, the distance being around 100 light years, while Paul Scott and his student R. A. Collins made observations with a separate telescope, with its own receivers, which eliminated instrumental effects. Having found no satisfactory terrestrial explanation for the pulses, they now began to believe that they could only be generated by some source far beyond the solar system; the short duration of each pulse suggested that the radiator could not be larger than a small planet. In his 1975 Nobel lecture, Hewish says, “We had to face the possibility that the signals were, indeed, generated on a planet circling some distant star, and that they were artificial. I knew that timing measurements, if continued for a few weeks, would reveal any orbital motion of the source as a Doppler shift, and I felt compelled to maintain a curtain of silence until this result was known with some certainty. Without doubt, those weeks in December 1967 were the most exciting in my life.”
As Bell recalled: “We did not really believe that we had picked up signals from another civilisation, but obviously the idea had crossed our minds and we had no proof that it was an entirely natural radio emission.” She was very worried: “... here was I trying to get a Ph.D. out of a new technique, and some silly lot of little green men had to choose my aerial and my frequency to communicate with us.” During the following days, in analysing a recording of a completely different part of the sky, amongst a strong, heavily modulated signal from Cassiopea A, she thought she saw some scruff. She rapidly checked through previous recordings of that part of the sky, and on occasions there was scruff there. Knowing that the scruff would transit in the early hours of the morning, she went early in the morning to the laboratory, and she found that this scruff too was a series of pulses, this time 1.2 seconds apart. She left the recordings on Hewish's desk and went off, much happier, for Christmas: “It was very unlikely that two lots of little green men would both choose the same, improbable frequency, and the same time, to try signalling to the same planet Earth.” Over Christmas, Hewish kept the survey running, put fresh paper in the chart recorders, ink in the ink wells, and piled the charts, unanalysed, on Bell's desk. When she returned after the holiday, she immediately settled down to do some chart analysis. Soon, on the one piece of chart, an hour or so apart in right ascension, she saw two more lots of scruff. It was another fortnight or so before another one was confirmed, and soon after that the third and fourth were also.
At the beginning of February 1968, the paper “Observation of a Rapidly Pulsating Radio Source”, announcing the first pulsar, was submitted to Nature. It was signed by Hewish, Bell, Pilkington, Scott, and Collins. By that time they were confident that three additional pulsars existed although their parameters were then only crudely known. They also mentioned that at one stage they had thought the signals might be from another civilisation. They suggested that the pulses might be generated by a white dwarf star, or, more likely, a hypothetical neutron star. The existence of neutron stars was first proposed by Walter Baade and Fritz Zwicky in 1934, when they argued that a small, dense star consisting primarily of neutrons would result from a supernova explosion. In the late 1930s, Robert Oppenheimer and his collaborators predicted that when a massive star died, it would collapse into an incredibly dense, spinning body, a neutron star. In 1967, shortly before the discovery of pulsars, Franco Pacini suggested that a rotating neutron star with a magnetic field would emit radiation. After the discovery of the first pulsar, Thomas Gold independently suggested a rotating neutron star model similar to that of Pacini and explicitly argued that a pulsar is accompanied by an enormously powerful magnetic field surrounded by a plasma cloud, emitting a rotating beam. This model could explain the pulsed radiation observed by Bell and Hewish. When Stephen Hawking heard their news he was delighted and phoned Hewish to say that if neutron stars existed then black holes were almost certain to occur also. The detection of pulsed light from the star that had previously been identified as the remnant of the original explosion, observed in 1054 A. D., provided further impressive support for the neutron star hypothesis. This, according to theories of stellar evolution, was precisely where a young neutron star should be created.
By 1968 most opinion settled on neutron stars as the best solution for pulsars. These extremely dense stars, which form from the collapsed remnants of massive stars after a supernova, have strong magnetic fields that are not aligned with the star’s rotation axis. They spin very rapidly, up to nearly 1000 times per second. The strong field and rapid rotation produces a beam of radiation that sweeps around as the star spins. Jets of particles moving almost at the speed of light are streaming out above their magnetic poles. These jets produce very powerful beams of light and their radio emission is concentrated along a narrow cone. For a similar reason that “true north” and “magnetic north” are different on Earth, the magnetic and rotational axes of a pulsar are also misaligned. Therefore, the beams of light from the jets sweep around as the pulsar rotates and on Earth, we see this as a series of pulses, much like the beacon from a rotating lighthouse lamp. Pulsars are formed with a certain amount of angular momentum. As gravity causes them to shrink (and thus have a smaller radius) they must spin faster in order to conserve angular momentum.
In 1968, Bell earned her PhD - pulsars appeared in the appendix of her dissertation. It became soon clear that this discovery must rank as one of the great events in astronomy. Their unique properties made them nearly ideal probes for a wide range of physical studies, opening up new horizons in fields as diverse as quantum-degenerate fluids, relativistic gravity and interstellar magnetic fields. The outstanding observational characteristic of pulsars is the pulsed emission and its precise periodicity. This great stability provides the basis for many of the applications of pulsars to studies of physical phenomena. The neutron stars represent matter in bulk at nuclear densities and offer many challenges for physicists and astrophysicists.
In 1974, Antony Hewish and Martin Ryle became the first astronomers to be awarded the Nobel Prize in physics, with the Royal Swedish Academy of Sciences noting that Hewish had played a “decisive role in the discovery of pulsars”. By that time, more than 130 pulsars charted in the heavens, there was overwhelming evidence that the neutron star “lighthouse” model was correct. No other star could spin fast enough, without fragmenting. However, considerable controversy was associated with the fact that Hewish was awarded the prize while Bell, who played a pivotal role in the initial discovery while she was his Ph.D student, was not. Bell claimed no bitterness upon this point, supporting the decision of the Nobel committee.
That same year, using the Arecibo Observatory, Joseph Taylor, Jr. and Russell Hulse discovered for the first time a pulsar in a binary system, PSR B1913+16. This pulsar orbits another neutron star with an orbital period of just eight hours. Einstein's theory of general relativity predicts that this system should emit strong gravitational radiation, causing the orbit to continually contract as it loses orbital energy. Observations of the pulsar soon confirmed this prediction, providing the first ever evidence of the existence of gravitational waves. In 1993, the Nobel Prize in Physics was awarded to Taylor and Hulse for the discovery of this pulsar.
In PSR 1913+16 only one of the pair of neutron stars is observed as a pulsar, but in the binary system PSR J0737-3039, both neutron stars are observed as pulsars. This remarkable system discovered in 2003 at Australia's Parkes Observatory by an international team led by the radio astronomer Marta Burgay, makes it possible to determine the parameters of their binary orbits very precisely. The binary period about the centre of momentum of the system is only 2.4 hours, the shortest yet known for such an object (one-third that of the Taylor-Hulse object). The effects of general relativity are even stronger than in PSR 1913+16, thus this binary neutron star system can provide some of the most stringent tests of general relativity. Radio observations from Australia, Germany, England, and the United States show that as a result of energy loss due to gravitational waves the orbit shrinks by 7 millimetres per day, exactly in accordance with what Einstein's theory predicts. The two components will coalesce in about 85 million years.
Bibliography
Bell Burnell J. Little Green Men, White Dwarfs or Pulsars? Cosmic Search 1 (1), http://www.bigear.org/vol1no1/burnell.htm
Interview With Antony Hewish, 1974 Nobel Prize Laureate In physics, By Kourosh Ziabari. 17 October, 2012, http://www.countercurrents.org/ziabari171012.htm
Hewish A. (2001) Prelude to Discovery. The Kenyon Review 23 (2): 147-157
Hewish A. (1974) Pulsars and high density physics. Nobel Lecture. http://www.nobelprize.org/nobel_prizes/physics/laureates/1974/hewish-lecture.pdf
Hewish A. interviewed by A. Smith. June 2009. http://www.nobelprize.org/nobel_prizes/physics/laureates/1974/hewish-interview.html
Longair M. (2011) The Discovery of Pulsars and the Aftermath. Proceedings of the American Philosophical Society 155: 147-157