Prof. Dr. Frédéric Joliot-Curie > Research Profile
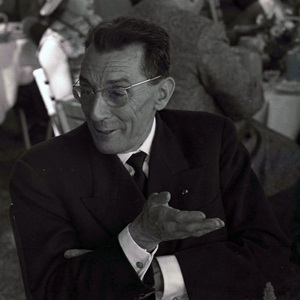
by Luisa Bonolis
Frédéric Joliot
Nobel Prize in Chemistry 1935
"in recognition of their synthesis of new radioactive elements".
Becoming Assistant to Marie Curie at the Radium Institute
Frédéric Joliot was born in Paris, on 19 March 1900. After his school studies, he decided upon a scientific career and was admitted to the École Supérieure de Physique et de Chimie Industrielle of the city of Paris, where his interests gradually gravitated to basic scientific research under the decisive influence of one of his teachers, the prominent French physicist Paul Langevin. During these years a large portion of the curriculum was devoted to laboratory work, so that Joliot had the possibility to develop his talents as an experimenter, too.
After graduating first in his class with a degree in engineering, Langevin advised him to apply for a position at the Radium Institute of the University of Paris, directed by Marie Curie, and in 1925 Joliot became her personal assistant. There he served as a preparator, and at the same time he undertook further studies in the chemical properties of radioactive elements.
When Joliot entered the Radium Institute, Pierre and Marie Curie's elder daughter, Irène, had already been an assistant there since the end of World War I and had begun her own research in 1921 on the radioactivity of polonium, the element discovered by her parents in 1898, along with radium. For their researches on the new radioactive bodies the Curies had been awarded the 1903 Nobel Prize in Physics, that they shared with Henri Becquerel, the discoverer of the phenomenon of natural radioactivity.
Within a year, in October 1926, Irène and Frédéric were married. It was at that time, to express their intimate collaboration at work more clearly, that they came to be known as Joliot-Curie and from 1928 onwards their names appeared frequently in papers to the Académie des Sciences.
After concluding his first personal research on the electrochemical properties of polonium, Joliot obtained his Ph.D. in 1927 and received one of the first scholarships from the Caisse Nationale des Sciences, which enabled him to remain at the Institute du Radium. He pursued his research on the effects of radiation and at the same time he designed and supervised the construction of a greatly improved cloud chamber, an equipment that could function at various pressures and would thus enable him to better observe the ionizing radiations emitted directly or secondarily by radioactive substances. The cloud chamber could operate in a magnetic field of 1,500 gauss, produced by large coils surrounding the cylindrical chamber in which the expansion took place. This arrangement permitted Joliot to determine the energy of the beta-rays by measuring the curvature of their trajectories on the photographs. In collaboration with his wife Irène, who had specialized for many years in the study of polonium, he prepared very strong sources of alpha particles emitted by this element, a main tool for probing atomic structures since the pioneering studies performed by Ernest Rutherford. By the end of 1931 they had the most important stock of polonium in the world.
A Mysterious Radiation from Beryllium
In the four years following their marriage, the Joliot-Curies built up their scientific and technical resources, so they were ready to accept the challenge offered by an intriguing phenomenon discovered in 1930 by the German physicists Walther Bothe and Herbert Becker. They had found that certain light elements, notably beryllium and boron, exposed to a bombardment of alpha particles emitted a very penetrating radiation which was able to pass through 10 cm of lead without a real fall-off in intensity. They though it to be a very short-wave electromagnetic radiation of hard X-rays. Like other physicists, the Joliot-Curies were attracted to the questions this discovery posed, and at the end of 1931 they began to study the mysterious radiation, using their own intense source of alpha-particles from their very strong polonium preparations and placing it against a beryllium plate. They used both their highly sensitive ionization chamber to measure its intensity and a cloud chamber to obtain photographs. To be sure that they were dealing only with the very penetrating radiation they first passed it through 15 mm of lead before allowing it to enter the ionization chamber, which they had sealed with a very thin layer of aluminum, only five thousandths of a millimeter thick.
If the Bothe-Becker radiation, in passing through various substances, produced a secondary radiation that was not highly penetrating and easily absorbed, this, too, could be revealed by the ionization chamber. In front of this aluminum sheet they were able to interpose plates of various substances. The current through the chamber remained generally constant, but they actually observed a marked increase of ionization in the chamber when the penetrating radiation collided with a screen containing hydrogen (such as paraffine wax, water or cellophane). It was a most surprising result, meaning, surely, that something else was being produced, which could not have entered the chamber if the wall had been a fraction (a few tenths of a millimeter) thicker. This led them to conclude that the Bothe-Becker radiation was capable of knocking protons out to the wax, setting them in rapid motion. After measuring the range of these protons they calculated that they must have an energy of 5.3 MeV. Assuming they were produced in the head-on collision of a gamma-ray photon with a proton, they came to the view that the Bothe-Becker radiation consisted of gamma-rays having an energy at least of 50 MeV. This was about ten times as energetic as any previously known nuclear gamma radiation, and in fact, when James Chadwick reported their experiments to Rutherford, the latter exclaimed: “I don't believe it.” Experiments on the Bothe-Becker radiation were followed with great interest also in the Physics Institute of Rome University. According to members of Fermi's group, as soon as Ettore Majorana heard about the Joliot-Curies' experiments, he criticized their interpretation, and observed that they had discovered the “neutral proton” without being aware of it.
In his most famous Bakerian Lecture of 1920, Rutherford had actually argued that one nuclear electron might bind one proton, producing an “atom” of unit mass and zero charge, whose existence seemed “almost necessary to explain the building up to the nuclei of heavy elements.” Soon after, Rutherford moved to the Cavendish Laboratory, and encouraged attempts to reveal such a neutral particle, but no experimental evidence for its existence could be obtained for years. James Chadwick, working at Cavendish Laboratory and since the beginning an enthusiastic believer in the existence of the neutron, thus had a prepared mind.
As soon as he read the Joliot-Curies' first note presented to the Academy of Sciences on 18 January 1932, he became convinced that their observations could not be ascribed to a kind of Compton effect, and that the beryllium radiation behaved very differently from a quantum radiation. The property of setting in motion the atoms of matter in its path suggested instead that the radiation consisted of particles. He began to study the ejection of atomic nuclei by Bothe-Becker radiation, employing a proportional pulse amplifier that allowed him to compare the energies of the ejected helium or nitrogen nuclei with that of the protons. A short period of strenuous work was sufficient to conclude that these ejections were the result of collisions between the nuclei and fast-moving uncharged particles that possessed a mass of the same order of magnitude as that of the protons and undoubtedly were torn from the nuclei of beryllium or boron by the alpha particles. He called this uncharged particle neutron and suggested that it was made up of a proton and an electron in close combination, further proposing that this particle was an essential part of atomic nuclei. The possible existence of a new nuclear constituent was put forward in a short letter to Nature on 27 February 1932. Chadwick actually began his paper by acknowledging that the first experimental discoveries which had laid a serious basis for the hypothesis of the existence of the neutron were the works of the Joliot-Curies.
The latter recognized that they had missed a major discovery, but they continued their researches giving a precise determination of the mass of the neutron at rest, from which they deduced that the neutron must be heavier than the proton and hence unstable, being able to decay into a proton and electron. They also reported that when beryllium is bombarded with polonium alpha particles, both neutrons and gamma rays are emitted simultaneously.
In detecting the recoil protons in their ionization chamber and photographing their tracks after entering Joliot's cloud chamber immersed in a 1500-gauss magnetic field, they also observed tracks of electrons expelled from a lead filter, one of which was “curved in an opposite sense to the others.” In the early 1930s, the only subatomic particles known were electrons, protons and neutrons. There was also the photon, a “particle” without mass associated with the electromagnetic radiation. In that same year, the U.S. physicist Carl Anderson, after studying thousands of tracks obtained in a cloud chamber divided in two by lead, found a particle whose curvature indicated that it had a mass equal to that of the electron, but curving in the opposite direction. The existence of the positive electron was brilliantly confirmed by Patrick Blackett and Giuseppe Occhialini in England, who used a most efficient cloud chamber whose expansion was triggered by particles entering the chamber through an electronic coincidence circuit devised by the Italian physicist Bruno Rossi. With this brand new device, with which particles took photographs by themselves, Blackett and Occhialini were able to demonstrate the appearance of a double track, which was due to the formation of an electron-positron pair within the ceiling of the chamber, thus confirming P.A.M. Dirac's relativistic quantum theory of the electron, which also implied the existence of antimatter.
The Joliot-Curies realized they had photographed the track of a positron only after learning of these experiments. But they were able to produce the first ever recorded photograph of the creation of an electron-positron pair due to the materialization of a gamma photon in a cloud chamber, a work which actually became the stepping stone to their great discovery.
The Discovery of Artificial Radioactivity
In fall 1933, after the seventh Solvay Physics Conference held in Brussels on the “Structure and properties of atomic nuclei,” Irène and Frédéric were working independently on different researches. Joliot in particular continued with experiments on the properties of the “materialization positrons” proving that annihilation is accompanied by the emission of gamma photons, which indeed nearly all had an energy of about mc2, that is about 500 keV, in complete agreement with Dirac's theory.
Resuming his investigations on the emission of neutrons and positive electrons by aluminum bombarded by alpha particles, Joliot covered the window of his cloud chamber with a thin sheet of aluminum foil, against which he placed a strong source of polonium. Much to his surprise, he discovered that, whereas the emission of neutrons ceased immediately after the polonium alpha-particle source was removed, the expected emission of positive electrons induced by the polonium, continued for several minutes decreasing exponentially with a half-life of 3 minutes and 15 seconds, after all irradiation of the aluminum had ceased. Joliot immediately called his wife and together they verified that the radioactivity was analogous to the beta radioactivity of the natural radioelements, but was of a new type, since the electrons emitted were positive. The formation of atoms emitting delayed positive electrons appeared to be associated with the emission of neutrons, which had already been previously observed, according to the nuclear reaction: aluminum-27 + helium-4 → phosphorus-30 + neutron. These atoms therefore had to be atoms of a radioactive isotope of phosphorus which would then decay with the emission of a positron transforming into atoms of one of the known stable isotopes of silicon: phosphorus-30 → silicon-30 + positron. They had similar results with boron, which transformed into a radioactive isotope of nitrogen.
From a physical point of view, these were convincing results, but it was necessary to test whether the active elements were chemically distinct from original nuclei.
Through several chemical experiments, in which their skill was utilized in the chemical identification of minute amounts of active elements (on the order of 10-16 grams), the Joliot-Curies were able to provide the first chemical proof that the element formed by transmutation was different from the original element.
Irène Curie and Frédéric Joliot announced the discovery of a new type of radioactivity and of the artificial formation of light radioelements in a note to the Academy of Sciences on 15 January 1934. The next February 10 a short paper appeared in Nature, where they remarked that: “positrons of aluminum seem to form a continuous spectrum similar to the beta-rays;” in this case, it thus appeared necessary to admit the simultaneous emission of a neutrino (or of an antineutrino) “in order to satisfy the principle of the conservation of energy and of the conservation of the spin in the transmutation.”
The next year, in November 1935, the couple was awarded the Nobel Prize in chemistry “for their synthesis of new radioactive elements.” Joliot was 35 years old, to date the youngest to receive the Nobel Prize.
The possibility of artificially creating radioisotopes of know stable elements aroused an enormous interest all over the world. All kinds of radioactive materials could be made, and applied widely in biology, medicine, industry and throughout the sciences and engineering.
When Irène and Frédéric presented to Marie Curie the first chemically isolated artificially radioactive element, an intense expression of joy and satisfaction seized her in taking between her fingers, burnt and scarred by radium, the small tube containing the feeble active material. She died soon after, the following July, before she could share with the couple the joy of the great new honor bestowed upon their family.
In the meantime their experiments had been repeated and extended in the major nuclear physics laboratories. In Rome, Enrico Fermi succeeded in obtaining artificial radioactivity using neutrons and extended bombardment to most elements, even those of high atomic mass, like uranium and thorium. Fermi's group assumed that in this case transuranic elements had been produced, thus giving rise to the famous “uranium puzzle” which was eventually solved by the chemists Otto Hahn and Fritz Strassmann working in Berlin. A new nuclear process was thus revealed which was named fission by Otto R. Frisch and Lise Meitner, who provided the physical explanation of the phenomenon: the nucleus of a uranium atom can be split into two nuclei of similar mass under the action of neutrons. Joliot immediately furnished a direct physical proof of the explosive character of the process which independently was established in Copenhagen by Frisch. Joliot also succeeded in taking the first cloud photograph of a fission fragment.
In becoming aware of the principle of the liberation of the internal energy of uranium atoms and of the conditions for the development of the nuclear chain reactions, physicists were inaugurating the modern nuclear era, which would soon deeply change for ever the human condition.
Bibliography
Bensaude-Vincent B. (1993) Frédéric Joliot. In K.J. Laylin (ed.), Nobel Laureates in Chemistry. 1901-1992. American Chemical Society and the Chemical Heritage Foundation, pp. 217-222
Blackett P.M.S. (1960) Jean Frédéric Joliot. Biographical Memoirs of Fellows of the Royal Society 6: 86-105
Goldsmith M. (1976) Frédéric Joliot-Curie. Lawrence and Wishart, London
Guerra F., M. Leone, N. Robotti (2012) The Discovery of Artificial Radioactivity. Physics in Perspective 14: 33-58
Perrin F. (1981) Joliot, Frédéric. In Dictionary of Scientific Biography, Vol. 7. Gillispie C.C. (ed.), Charles Scribner's Sons, New York, pp. 151-157