Prof. Dr. Irène Joliot-Curie > Research Profile
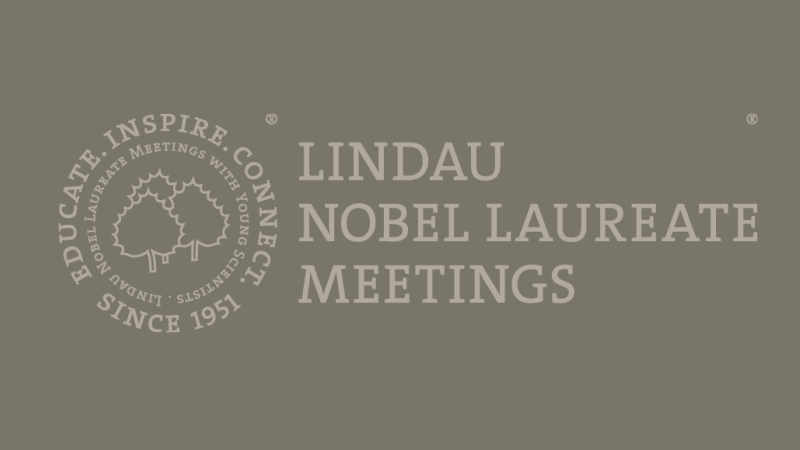
by Luisa Bonolis
Irène Curie
Nobel Prize in Chemistry 1935
"in recognition of their synthesis of new radioactive elements".
Following Family Traditions
Irène Curie, the first of two daughters of Pierre Curie and Marie Sklodowska, was born in Paris in 1897, at the turn of the century, when most of the basic ideas held by scientists were on the verge of being overturned. Only two years earlier, Wilhelm Conrad Röntgen had discovered a new kind of radiation which he called X-rays, and soon after Henri Becquerel found out by chance that the salts of uranium spontaneously emanated another new type of radiation - a radiation that could pass through metal foil and darken a photographic plate. Soon after Irène's birth, Marie Curie chose the mysterious uranium rays as a subject for her doctoral thesis and soon her husband Pierre joined her in a fruitful collaboration, during which they played a major role in opening up the science of radioactivity, a term Marie herself coined. Irène was only one year old when Marie and Pierre announced the existence of two new radioactive elements, radium and polonium, and she was six when her parents were awarded the Nobel Prize in Physics 1903 in recognition of their pioneering joint researches on the new radiation phenomena. They shared the Prize with Henri Becquerel who had discovered the phenomenon of spontaneous radioactivity.
After Pierre Curie's tragic death in 1906, Marie, who was the first woman to be awarded a Nobel Prize, was given Pierre's Sorbonne chair, thereby also becoming the first female professor in France. At that time Irène began studying at a cooperative school organized by her mother and several colleagues, who strongly disapproved of the rigid French educational system. Irène next went to the Collège Sévigné and graduated on the eve of World War I continuing her education at the Sorbonne, the University of Paris, where she took examinations for a license in physics and mathematics. During the war she served as an army nurse, helping her mother in setting up apparatus for the radiography of the wounded, and she also installed mobile X-ray units in military hospitals and trained personnel to operate them, receiving her first dangerous dose of radiation. In November 1911, she had accompanied her mother to Stockholm, when Marie Curie was awarded the remarkable distinction of a second Nobel Prize, in chemistry, for the discovery of radium and polonium and the preparation of pure radium.
After the war, Irène became a research assistant at the Radium Institute organized and managed by her mother. Following the research initiated by her parents, she investigated the fluctuations in the range of alpha-rays emitted in the disintegration of polonium and of gamma-rays emitted by radium. Both elements had been discovered by her parents more than twenty years earlier. She presented her doctoral thesis in 1925. Her early investigations involving alpha-particles (the very stable entities formed by two neutrons and two protons), also involved their use as a tool for probing atomic nuclei and elucidating their structure, according to the tradition inaugurated by Ernst Rutherford at the beginning of the century, which had led to the formulation of his nuclear model of the atom in 1911.
In October 1926, Irène Curie married Frédéric Joliot, a brilliant fellow assistant at the Radium Institute. It was the beginning of their joint scientific adventure, which combined personal love with social and political commitment, and especially the same devotion to scientific research which had characterized her parents' relationship.
Irène Curie was one of only a handful of experts in radioactivity during the 1920s, and her mother's Radium Institute was one of only a few world centres in the field. Building on Marie Curie's original stock, they produced the world's largest and most powerful supply of polonium, a precious source of alpha-particles, but also a highly toxic element, which they handled without worrying too much about possible dangers, too engrossed as they were in their work. They were scientific partners for the rest of Irène's life - each being a fitting complement to the other - and collaborated during their most productive years.
The Bothe-Becker Radiation and the Neutron: a Missed Discovery
In 1930 Walther Bothe and his student Herbert Becker found that certain light elements produced what seemed to be a high-energy gamma-ray emission when exposed to a bombardment of alpha particles from polonium. To their surprise, rays emerged from the beryllium with so much power that they could penetrate lead two centimetres thick. After some absorption measurements, the German physicists concluded that the penetrating power of the observed unusual radiation was of the same order as the hardest radioactive gamma-rays. Bothe was one of the most thorough experimental physicists of his time, who later received the 1954 Nobel Prize in Physics for his invention and study of the coincidence method, thus, for more than one reason, Irène Curie and Frédéric Joliot found the results of the German physicists especially challenging. It was the occasion for the beginning of their constant collaboration, lasting several years. They were going to compete with a handful of other nuclear physicists, including Ernest Rutherford and his collaborators in Great Britain, Lise Meitner at the Kaiser Wilhelm Institute in Berlin, and Niels Bohr in Copenhagen. But new unsuspected discoveries would very soon stimulate more physicists to enter this new promising field.
The French couple separately measured the absorption coefficient in lead of the penetrating Bothe-Becker radiation in the case of beryllium and lithium (Curie) and in the case of boron (Joliot). The next important step was contained in a paper which was presented to the Academy of Science on January 11, 1932. To study the new rays, Curie and Joliot placed their polonium source of alpha particles next to beryllium and observed the emission of powerful rays. Thinking that photons of such high energy could perhaps produce some kind of transmutation, they placed thin layers of various substances in contact with the upper wall of their ionization chamber, which consisted of a thin aluminium foil. While no change in the ionization current was observed in all other cases studied (C, Al, Cu, Ag, Pb), in the case of paraffin (or of other hydrogenous substances, such as water or cellophane) an increase by about a factor of two was observed. After some experiments they concluded that paraffin was ejecting high-energy protons under the action of the Bothe-Becker radiation. In order to explain the production of recoil protons by what they considered high-energy gamma rays, Curie and Joliot thought first that the observed phenomenon was perhaps similar to the Compton effect, in which photons impinging on a metal surface eject electrons. By applying the laws of energy and momentum conservation, they thus concluded that the photons emitted from beryllium and boron must have an energy of at least 50 and 35 MeV, respectively. Later they realized the serious difficulties involved in such an interpretation and in a later paper they put forward the assumption that the observed effect was due to a different type of interaction between gamma-rays and protons.
But in the meantime, their remarkable result had been reported to Rutherford by his collaborator James Chadwick, who in particular did not share their opinion that the observations should be ascribed to a kind of Compton effect, because he had looked for this more than once and had thus thoroughly investigated the problem. He was on the contrary convinced that there was something quite new and strange going on. Chadwick repeated the Joliot's experiments in Rutherford's laboratory with some polonium from Lise Meitner, and began his own experiment with an open attitude, but with the idea of a heavy neutral particle in mind. Since his famous Bakerian Lecture of 1920, Rutherford had conjectured the existence of a neutral nuclear constituent which would explain the disparity found between the atomic number of an atom and its atomic mass. However, he considered the neutron, as it was called by him and his collaborators, to be a close combination of a proton and an electron, an idea which continued to be generally accepted through the 1920s and early 1930s. Since then, many experiments had been done to look for Rutherford's neutron at Cavendish Laboratory in Cambridge, but in vain. Chadwick, who had a long experience in nuclear physics, had now more than one good reason to believe that the Joliot-Curie experiments had somehow produced an experimental proof of its existence, and that they had misinterpreted their data.
In his experiment Chadwick used as detector an ionization chamber connected to a linear amplifier, a crucial device for this kind of experiment: the ionization produced by a single heavily ionizing particle could be measured. With this apparatus Chadwick proved that the secondary penetrating radiation emitted from beryllium could produce recoil atoms not only from hydrogenous substances but also from helium, lithium, beryllium, air and argon. In the case of nitrogen, he also measured the range of the recoiling atoms to make sure that it remained completely within the ionization chamber. He was able to reach the conclusion that the kinetic energies transferred to the atoms of hydrogen and nitrogen did not correspond to the same energy of the primary radiation, if this was composed of photons. Chadwick also applied conservation laws to the collision process, measuring the momenta transferred to different atoms. The conclusion of both arguments was that the interpretation of the observations in terms of photons was unacceptable. But all difficulties disappeared and the observed effects could be fitted into a satisfactory picture under the assumption that the beryllium nucleus, bombarded with alpha particles, emitted a neutral particle of mass very close to that of the proton. These experiments and arguments showed also that this new particle could be produced in light elements through what are called (alpha, n) processes, which in the case of beryllium gave a nucleus of carbon-12 and a neutron according to the reaction: helium-4 + beryllium-9 --> carbon-12 + n.
The possible existence of a new nuclear constituent was put forward in a short letter to Nature on 27 February 1932. Chadwick actually began his paper by acknowledging that the works of the Joliot-Curies had supplied the decisive clue for this discovery. Chadwick was awarded the 1935 Nobel Prize for physics, for detecting the neutral constituent of the nucleus foreseen by Rutherford, an achievement which began the science of nuclear physics in earnest and opened the way to modern theories of the nuclear structure.
Neutrons, Positrons and the Discovery of Artificial Radioactivity
Curie and Joliot had missed a major discovery, but they continued their researches giving a precise determination of the mass of the neutron at rest, from which they deduced that the neutron must be slightly heavier than the proton. In April 1932, while investigating the Bothe-Becker radiation using the cloud chamber designed by Joliot, they had found that boron and aluminium irradiated with alpha particles not only emitted protons and neutrons, but also generated electronic tracks in the cloud chamber. Some swift electrons in particular showed a curvature opposite to that of the others and thus seemed to be moving toward the source. Like many other experimenters since the early 1920s, who had not devoted enough attention to the phenomenon, they had actually recorded what turned out to be the tracks of positive electrons, which had appeared as theoretical entities in P.A.M. Dirac's theoretical papers published between 1928 and 1931. It was the US physicist Carl Anderson who explicitly identified the backward electron tracks with a positive particle of unit charge and of mass small compared to that of proton in an article published in September 1932. In March 1933, Anderson published a cloud-chamber photograph of what became the first known antiparticle and named the new elementary particle "positron." The photograph became an icon of XX century particle physics. Soon after his first article, Patrick Blackett and Giuseppe Occhialini fully confirmed Anderson's observation, explicitly relating it to the anti-electron hypothesis contained in Dirac's theory of the electron. They worked with a cloud chamber placed between two Geiger counters, working in coincidence, so that events were only recorded when both counters were simultaneously activated. In such a way, tracks of positive electrons occurring in showers were found in 80% of the photographs.
The Nobel Prize in Physics 1936 was awarded to Victor Franz Hess "for his discovery of cosmic radiation" and to Carl David Anderson "for his discovery of the positron".
Curie and Joliot once again felt that they had missed a new important discovery, and began to investigate the mechanism of the emission of positrons. According to Dirac's theory, an encounter between two hard gamma rays (of an energy of at least half a million volts) could lead to the simultaneous creation of an electron and an anti-electron. The origin of the positrons was conclusively established in spring 1933 by Curie and Joliot, who were soon able to communicate that in some of their photographs pairs of electron-positron tracks seemed to originate from the same point within the gas of the cloud chamber, an observation which led them to conclude that such electron-positron pairs appeared to be emitted simultaneously out of the interaction between high energy photons and the heavy lead nuclei. It was actually the first clear demonstration of the transformation of electromagnetic radiation into matter.
In fall 1933, after participating in the seventh Solvay Physics Conference held in Brussels on the “Structure and properties of atomic nuclei,” to which the most prominent nuclear physicists had been invited, Curie and Joliot resumed their investigations on the emission of neutrons and positive electrons from aluminium bombarded by alpha particles. In order to check the assumption that neutrons and positrons were emitted simultaneously, whatever the energy of the bombarding alpha-rays, in early January they began to irradiate aluminium with alpha particles and gradually decreased the energy with the aim of determining the minimum energy of alpha-rays provoking the emission. They were surprised to observe that in progressively reducing the energy of the alpha rays, the emission of neutrons ceased altogether when a minimum velocity was reached, while the positrons continued to appear, decreasing only over a period of several minutes, like the radiation of electrons from a naturally radioactive element.
After verifying that everything was in order with the Geiger-Müller counter, they realized that this phenomenon was quite different from all the others produced up to that time; all the induced reactions of nuclear chemistry were instantaneous phenomena, as in the case of the artificial transmutations performed by Rutherford and his collaborators, in which stable light elements were produced, or in the case of positrons emitted by boron when bombarded by alpha particles. But in the case of aluminium, the positive electrons produced under the action of alpha rays continued to be emitted for some time after removal of the source. Their number decreased by half in three and a half minutes. They well knew that sound: clicking of the Geiger and dying down gradually suggested a radioactivity phenomenon. They inferred that aluminium nuclei had absorbed alpha particles from the polonium, ejected neutrons, and, in the process, for a few short moments had changed into a heavier element, an artificial type of phosphorus. Because ordinary phosphorus atoms are not radioactive, they concluded that they had observed an unknown radioactive isotope of this light element. Such an isotope was so unstable, however, that it soon ejected the positrons and was quickly transmuted into a stable form of silicon. The decay of the activity was exponential: for irradiated boron and magnesium it was 14 and 25 minutes, respectively. Curie and Joliot had induced naturally stable elements to become artificially radioactive. It was a new type of radioactivity, in which positive electrons were emitted. They found that the distribution of the positrons was in a continuous spectrum, similar to the typical decay by electron emission (beta-negative), and this confirmed them in their idea that they had produced radioactive bodies analogous to the natural radioactive elements.
They began immediately to search for ways to prove chemically that the aluminium had indeed changed into phosphorus on its way to becoming silicon.They had only trace amounts of phosphorus, however, and half of it decayed within three and a half minutes. But Irène devised a three-minute chemical test: she dissolved a thin sheet of irradiated aluminium in hydrochloric acid. The hydrogen liberated was collected in a thin-walled tube which was found to carry the activity with it. As the decay was very speedy, the work had to be done in three minutes. This, and other chemical experiments with boron and magnesium, provided proof that the elements formed by transmutation were different from the original element. Since aluminium consists of a single isotope, they were able to conclude that, in this case, the alpha-particle was captured in the nucleus.
Irène Curie and Frédéric Joliot announced the discovery of a new type of radioactivity and of the artificial formation of light radioactive isotopes in a note to the Academy of Sciences on 15 January 1934. Later, after having isolated the first artificially radioactive element, they proudly gave Marie Curie a tiny test tube containing "the first atomic nucleus created by man." In holding it up to a Geiger counter and in hearing the characteristic sound, her face glowed with an intense joy. It was her last great satisfaction. Marie Curie died of acute leukemia in July 1934 and thus could not witness the triumph of her daughter and son-in-law, similar to her own accomplishment with Pierre Curie thirty-two years earlier.
Irène Curie and Frédéric Joliot were nominated for the physics Nobel Prize in 1934, but passed over that year, and in 1935 they maintained the brilliant family tradition being awarded the Nobel Prize in chemistry - the third Nobel Prize for the Curie family - "for the synthesis of new radioactive elements".
The appearance of Curie and Joliot's article in January 1934 had not escaped Ernest Lawrence's attention. He immediately realized that in his laboratory at Berkeley, the Geiger counter and the cyclotron worked simultaneously, so that they immediately arranged to have them operating independently and put a carbon target into the beam. When the cyclotron was deactivated, the crackling of the Geiger announced the presence of the first nitrogen-13 ever known to exist in the world. They looked at each other, all aware that they could have made the discovery at any time.
Curie and Joliot's discovery of artificial radioactivity was the result of about two years of experimental work of remarkable quality, which had paved the way to the discovery of the neutron. In opening the door to the production of radioisotopes of al known elements, it gave an enormous power to applied radiochemistry: radioelements were no more the object, but the tools of a research field which has gained an extraordinary importance since then. A new era was opening, not only in physics but in chemistry, biology, geology, and medicine.
Transuraniums: a Challenge and a Puzzle
In 1936 Irène Curie served for four months as Under-Secretary of State for Scientific Research in Leon Blum's Popular Front Government and was elected professor at the Sorbonne in 1937. This office and her numerous other duties related to the Radium Institute absorbed a great deal of her time and attention; moreover, her health was showing signs of having been affected by her exposure to X and gamma-radiations during World War I and then in the laboratory, when the dangers of working with strongly radioactive materials were still not fully realized. Like her mother, she would die at the age of fifty-eight, a victim of acute leukemia. But now, during the second half of the 1930s, she did her most remarkable individual work. Her attention was attracted by the problem of the artificial radioelements produced by the irradiation of uranium by slow neutrons which had been inaugurated by Enrico Fermi in Rome. Fermi's group performed extensive work which established that nearly every element can be made radioactive, if particles with sufficient intensity were available. After bombarding heavy elements like uranium and thorium, Fermi thought that neutron capture followed by beta decay, which left the target nucleus with one more unit of positive charge, had, in the case of the last known elements of the periodic table, led to the production of the transuranic elements Z=93 and Z=94. In Rome they even used to call such "new" elements Ausonium and Hesperium, after the names of ancient Italic people.
Starting from 1935 she published with Hans von Halban and Peter Preiswerk a series of works on the elements produced by irradiating thorium. Aided by her great experience in radiochemistry inherited from her mother, Curie sought to analyse the complex broth of elements and isotopes resulting from the bombardment. The substances making up the broth, all highly radioactive and transmuting into one another with varying half-lives, were too small in quantity to be discernible other than by their emitted radiations. Therefore, Curie and her collaborators resorted to the standard radiochemical procedure of adding a chemically related, stable isotopic "carrier" of the radioactive atoms. In this way, they extracted a sample mixture large enough to be manipulated, as the radioactive atoms followed the carrier through the chemical treatment.
In the meantime, the physicist Lise Meitner and the radiochemist Otto Hahn in Berlin had also taken up these studies and like Fermi had concluded that such products had to be transuranic elements of atomic number 93 or higher, adding to the growing consensus among scientists. Later, they continued to work along the path of the 'transuranium' decay series, following a blind alley.
The way out came from Paris. In 1937, after repeating the experiments of the Berlin team, Irène and her new collaborator, the Yugoslav physicist Pavel Savitch, made a change in the experimental arrangement that allowed them to perform longer irradiations and use thicker uranium sources. Instead of relying on chemical separation, they concentrated on whichever isotope gave off the most penetrating beta-rays. They were thus able to observe in particular a new 3.5 hour activity which was not known to Hahn's group. The chemical behaviour of such activity showed that it could not be a member of the Berlin's team transuranium series. In Spring 1938, Curie and Savitch stated that it had "properties similar to those of the rare earths," and concluded that it might be "either an isotope of actinium, or a new transuranium body." But this radioelement was not an isotope of actinium, since by adding lanthanum to the actinium extract and then separating it out again through fractional precipitations, the new radioelement was shown to follow lanthanum, its chemical properties therefore being closer to those of lanthanum. They definitely concluded that "this body could only be a transuranium element possessing the properties quite different from those of the other known transuranics, a hypothesis raising great difficulties for its interpretation."
In their last paper, published in September 1938, Curie and Savitch summarized their work saying that their radioactive element of 3.5 hours half-life produced in uranium irradiated with neutrons - probably a transuranium element - definitely appeared to have properties similar to those of lanthanum. This conclusion immediately triggered Hahn's interest. By that time, Lise Meitner had unfortunately left Germany after her position, being Jewish, had become very difficult after annexation of Austria by Germany, so that the testing of news from Paris started in the autumn without her, and communication was only maintained through correspondence.
In working only with a single activity, the French had actually found a way out of the Fermi-Hahn transuranium blind alley. They had achieved two important steps out in solving the uranium puzzle: Their 3.5 hour substance could not be fitted into the four-year old concept of the transuranium isomer series. What was most important, their substance had shown properties unusually similar to an element far below uranium. Unfortunately it was not a pure radioactive isotope, so that they could not prove that the whole activity was due to lanthanum.
The final work was done by the outstanding German radiochemist Otto Hahn, assisted by the very skilled analytical chemist Fritz Strassmann. They repeated and extended the experiments and by December 1938 were able to confirm that uranium irradiated by neutrons produces not only radioactive atoms possessing chemical properties very similar to those of the lanthanides, but also atoms of a radioactive isotope of barium. This proved that, as in the case of Chadwick and the neutron, work in Paris had opened the door to an important discovery. These results forced Hahn to abandon the schemes followed during four years and led to the final jump that contradicted all previous experience in nuclear physics. What they had thought to be radium isotopes, actually had the properties of barium, so that their decay series radium --> actinium --> thorium had to be renamed barium --> lanthanum --> cerium. Even if with great hesitation, as chemists Hahn and Strassmann had to put forward the evidence that under the action of neutrons the uranium nucleus appeared to have been split into fragments consisting of lighter elements of comparable mass, lying very far from uranium in the periodic table. This unsuspected phenomenon was immediately interpreted from a physical point of view by Lise Meitner and her nephew Otto R. Frisch, who worked at Bohr's Institute in Copenhagen. In particular, they estimated that the liberated energy corresponding approximately to the difference in packing fraction between uranium and the elements in the middle of the periodic system should be about 200 MeV. After a suggestion by a biologist, they introduced the term fission for the new process.
Hahn and Strassmann's article, published in January 1939, established that the phenomenon involved was a partition of the uranium nucleus into two nuclei with nearly the same mass, and confirmed that Irène Curie had come within a hair's-breadth of discovering nuclear fission. The event concluded the incredible saga of the uranium puzzle, which had been a great challenge for radiochemists and nuclear physicists for four years, when data and assumptions from both chemistry and physics had guided and misguided their progress. In marking the beginning of the nuclear era, this discovery was fated to coincide almost exactly with the outbreak of World War II, a circumstance that determined all future developments in the applications of nuclear energy, with a giant impact on humanity.
Bibliography
Bensaude-Vincent, B. (1993) Irène Joliot-Curie. In K.J. Laylin (ed.), Nobel Laureates in Chemistry. 1901-1992. American Chemical Society and the Chemical Heritage Foundation, pp. 223-227
Chadwick, J. (1956) Mme. Irène Joliot-Curie. Nature 177 (4517): 964-965
Guerra F., M. Leone, N. Robotti (2012) The Discovery of Artificial Radioactivity. Physics in Perspective 14: 33-58
Leone M., Robotti N. (2010) Frédéric Joliot, Irène Curie and the early history of the positron (1932-33). European Journal of Physics 31: 975-987
Mladjenovic M. (1988) The Defining Years in Nuclear Physics. 1932-1960s. Institute of Physics Publishing, Bristol and Philadelphia
Perrin F. (1981) Joliot-Curie Irène. In Dictionary of Scientific Biography, Vol. 7. Gillispie C.C. (ed.), Charles Scribner's Sons, New York, pp. 157-159