Prof. Dr. Linus Carl Pauling > Research Profile
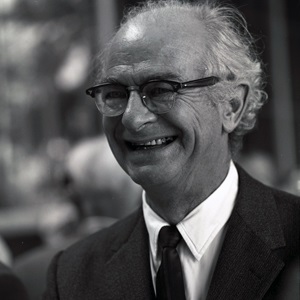
by Luisa Bonolis
Linus Carl Pauling
Nobel Prize in Physics 1954 and Peace Nobel Prize in 1962
Linus Carl Pauling, often called the Einstein of chemistry, is certainly one of the greatest scientists of all time. At 31 he became the youngest person elected to the National Academy of Sciences and at 36 became head of the Department of Chemistry and Chemical Engineering at Caltech. Best known for his foundational work in theoretical chemistry, biochemistry and molecular biology, Pauling played a formative role in at least four major developments in twentieth-century science: the application of quantum physics to chemistry, the use of theories of chemical structure in biology, the construction of molecular models, which became a characteristic tool of modern chemistry, the study of diseases as a product of molecular processes. He was nominated for a Nobel Prize in Chemistry almost every year from 1940 onwards, for different aspects of his wide-ranging research, and was awarded the Prize in 1954 “for his research into the nature of the chemical bond and its application to the elucidation of the structure of complex substances.”
Strongly encouraged by his wife Ann Helen Miller to become deeply aware of the social responsibility of scientists, Pauling, along with Albert Einstein and Leo Szilard, was a member of the Emergency Committee of Atomic Scientists, a group that after World War II worked to limit the spread of atomic weapons. Together with his wife, Pauling was a political activist for civil rights, nuclear disarmament, and world peace. His efforts to bring about world peace and the banning of nuclear weapons testing led to political persecution during the McCarthy era, including a 24-year investigation by the FBI. His passport and his government security clearance were revoked, and he was denied research grants. Although his peace activism damaged his reputation as a scientist among certain people and institutions, he considered these activities among the most important of his contributions to humanity. His active opposition to nuclear testing played an influential role in eventually bringing about the 1963 international treaty banning atmospheric tests. With the award of the 1962 Nobel Prize for Peace, Pauling became the first person to receive two unshared Nobel awards. This makes him one of only four individuals to have been awarded more than one Nobel Prize (the others being Marie Curie, John Bardeen, and Frederick Sanger). Pauling is also one of only two people to be awarded Nobel Prizes in different fields, the other being Marie Curie.
Becoming a Crystallographer
Linus Pauling was born in Portland, Oregon, in 1901. After attending Washington High School he entered Oregon Agricultural College in Corvallis, where he quickly attracted the attention of his teachers in his chemical engineering major. After his junior year, they enlisted him to teach freshman- and sophomore-level chemistry courses while he was still a student. One of his students, Ava Helen Miller, became his wife in June 1923. As he prepared his chemistry lectures in 1920, Pauling ran across Irving Langmuir's just published articles on the structure of atoms and the electron theory of the valence bond that stimulated him to read Gilbert Lewis’s 1913 paper, where the latter proposed the electron pair as the fundamental chemical bond, with the loss or capture of electrons accounting for chemical reactivity. At that time Pauling developed the belief that atomic arrangements are responsible for the chemical and physical properties of material substances. His keen interest in molecular forms and structure, which would guide his lifetime of research, led him to follow a course on the crystallography of metals. In 1912, the German physicist Max von Laue had put forward the idea that atomic sheets were about the right size to act like the slits in a diffraction grating for X-rays, as the space between them was of the same order of magnitude as their wavelength, much shorter than the size of visible light. His prediction was soon confirmed by firing a beam of X-rays at a crystal of zinc sulphide and capturing the scattered radiation on a photographic plate. When the plate was developed, there was a circular pattern of light and dark spots, a diffraction pattern. This proved that X-rays behaved like waves, settling a controversy that had lasted since their discovery, 17 years earlier. Max von Laue was awarded the 1914 Nobel Prize in Physics “for his discovery of the diffraction of X-rays by crystals”.
Henry and Lawrence Bragg, father and son, immediately pioneered the new technique, which involves a lot of mathematical analysis, and were able to determine the internal architecture of some crystalline solids, and thus to explain their properties. Once they had a beam of a specific wavelength, researchers could use X-rays to study the spacing of the crystal gratings: X-ray crystallography became the first probe for “seeing” the three-dimensional structure of matter at the atomic level. The first crystal structure the Braggs solved was rock salt. It was surprising to find that there were no discernible individual molecules of sodium chloride, contrary to what all chemists thought at the time. The crystal was one gigantic latticework, each sodium ion surrounded by six equidistant chlorides, each chloride by six equidistant sodiums. Another success was the discovery of the structure of diamond, pure carbon with its atoms linked, as earlier chemists had theorised, to form three-sided pyramids, tetrahedra. The infinite array of carbon atoms bonded strongly to others in all three dimensions explained its hardness. The Braggs shared the 1915 Nobel Prize in physics “for their services in the analysis of crystal structure by means of X-rays”. At 25, Lawrence Bragg is still the youngest ever recipient of the Nobel Prize.
Since modern crystallography began with X-ray diffraction experiments on crystals, the discipline has informed almost every branch of science by providing a means to understand the structure of complex molecules and materials. In the fall of 1922, when Pauling entered the California Institute of Technology, X-ray crystallography was still in its infancy, but laboratories in Europe were already describing the atomic architecture of dozens of crystals. He learned the difficult art of using the X-ray instrument, how to grow his own crystals, and how to prepare them and capture the X-ray diffraction patterns on photographic plates, measure the intensity and position of each important point, and analyse the patterns mathematically to see what they said about the atomic structure. By the time he was awarded his PhD, Pauling had published several papers, most on inorganic crystal structures, establishing a reputation as a promising young crystallographer. He completed his PhD in 1925 with the dissertation “The determination with X-rays of the structures of crystals.”
The Nature of the Chemical Bond
During his phD studies Pauling became aware of the great amount of existing knowledge in mathematics, physics, and chemistry, and he strove to become familiar with as much of it as possible. He followed lectures on mathematics, physics, and physical chemistry, on statistical thermodynamics and relativity theory, and on quantum theory with two of Europe's leading theoreticians, Paul Ehrenfest and Arnold Sommerfeld, both of whom visited Caltech during Pauling's student years. Pauling also collaborated with Peter Debye, who visited Pasadena in 1925, on a paper on the theory of solutions.
Pauling realised that in order to unravel the secret of molecular structure he would have to understand the nature of the atoms that made up the molecules. He immersed himself in physics, learning everything he could about the fast-changing theories of atomic structure. When he was awarded one of the newly established Guggenheim Fellowships to pursue postgraduate research, he of course chose to visit Europe. New revolutionary ideas were coming from European institutes of theoretical physics, mostly from young people like Wolfgang Pauli, Werner Heisenberg, Paul Dirac. All of them were born, like Pauling, between 1900 and 1901. In his application, he had proposed to apply the just discovered quantum mechanics to the problem of the structure of molecules and the nature of the chemical bond. He left the U.S. in the spring of 1926 and spent a year with Arnold Sommerfeld in Munich, whose institute was a centre for the development of new ideas about atomic structure, a month in Copenhagen with Niels Bohr, and six months in Zürich with Erwin Schrödinger, whose electron wave theory and equation had just appeared.
When he arrived in Munich in April 1926, the Bohr-Sommerfeld model was being displaced by the new quantum mechanics. Pauling was lucky to be at one of the centres of the new physics and thus he concentrated on learning as much as he could about the new theories. Pauling saw at once the implications of the new quantum mechanics for chemistry and how the new physics was destined to provide the theoretical basis for understanding the structure and behaviour of molecules. He discovered that Schrödinger's formulation afforded a simple and satisfactory atomic model, closely related to the chemist's atom. The wave function provided information about electron density about the nucleus, and thus about the shape and size of the atom. From his habit of building physical models of atoms and molecules, and from his thorough knowledge of empirical data on the sizes of atoms as could be obtained by X-ray diffraction studies, he now possessed a theoretical technique for calculating the size and shape of an atom. The data of X-ray crystallography could furnish independent checks on the accuracy of his solutions of the Schrödinger's equation. On the other hand, theoretically derived conclusions about the sizes and shapes of ions could be used to tackle the analysis of new and more complex crystals by X-ray techniques. The two approaches could complement one another to yield useful information about the nature of chemical bonds. His publications of 1927-1928 impressed Lawrence Bragg, who considered Pauling's work a landmark revealing the fundamental principles underlying all inorganic crystals.
Among the friends Pauling made in Munich were two young German physicists who shared his interest in the chemical bond: Fritz London and Walter Heitler. In the summer of 1927, they wrote a seminal paper on the hydrogen molecule in which they applied Werner Heisenberg's new notion of exchange or resonance energy arising from the interchange of two electrons with opposite spin. Combining this idea with Schrödinger's wave equation, Heitler and London extended wave mechanics to the level of molecules and were able to calculate the bond between two hydrogen atoms. When his European sojourn came to an end in spring 1928, Pauling had become an enthusiastic advocate for the ideas of Heitler and London, whose treatment of the hydrogen molecule became a prototype for Pauling's later treatment of more complex systems. He was now the only chemist to hold a first rank place among the scientists who applied quantum mechanics to the molecule in the interwar era. The structures of atoms, molecules, and crystals were since then central to his work.
By the time he returned to Pasadena to join the chemistry faculty at Caltech in the fall of 1927, he was only 26 years old, but had already turned out to be a prodigy. He worked hard to extend Heitler and London's ideas to more complex molecules. He clearly showed chemists how the new quantum mechanics could answer questions about the structure, magnetic properties, and bond strengths of molecules. His semi-empirical approach was based on application of Schrödinger's wave mechanics, on structural data from X-ray crystallography, laboratory results from chemistry and his own extraordinary ability to store incredible amounts of information about facts from chemistry, X-ray crystallography, mathematics, and physics. Based on this special blend, he developed his own characteristic style, using wave mechanics to clarify data about molecular structure from X-ray crystallography and vice versa. This interplay showed that Pauling was both an extraordinary crystallographer and an accomplished chemical theorist. All this helped him to communicate with chemists, who were not yet trained to tackle the difficult mathematics of quantum physics.
During the 1930s, his work on the nature of the chemical bond led him to formulate new concepts for which he himself introduced new terms that since then were associated with his name: hybridisation, resonance, and electronegativity. With these simple principles he explained how the structures and energies of molecules could be discussed to understand chemical phenomena.
In his lectures of the period 1929-1934, Pauling presented his notion of “mixed quantisation” of electron energy levels, later called hybridisation, setting up quantum wave functions to represent valence, or electron-pair, bonds, in carbon compounds. The theory of resonance of molecules among two or more valence-bond structures was based on the interchange energy of two electrons in his treatment of bond hybridisation. In resonance, the true state of a chemical system is neither of the component quantum states but some intermediate one, caused by an interaction that lowers the energy, thus making the actual normal state of the molecule more stable. Collaborating with George Wheland, Pauling explained aromatic compounds such as benzene, which had been studied since the nineteenth century. These puzzling molecular structures were conjugated molecules of alternating single and double bonds that Pauling explained as an instance of resonance. Using quantum mechanical principles, Pauling showed that these molecules can be represented by a linear combination of wave functions. As Pauling himself explained in his review of 50 years of progress in structural chemistry and molecular biology, the benzene molecule is “resonating among the several valence-bond structures.”
The many structures determined at Caltech helped Pauling to find a way of assigning to elements certain numbers that represented the particular atom's power of attracting electrons in a covalent bond. The so-called electronegativity scale was promptly adopted by chemists to determine the amount of ionic and covalent character in various chemical bonds.
A remarkable series of papers entitled The nature of the chemical bond formed the basis for his later book with the same title, where he summarised his quantum-mechanical ideas about how the new physics explained the chemical bond, how those bonds explained the structure of molecules, and how molecules' structure explained their behaviour. The first edition of his magnum opus The Nature of the Chemical Bond and the structure of molecules and crystals: an introduction to modern structural chemistry was published in 1939. This textbook changed the way scientists thought about chemistry, which now appeared as a discipline unified by an underlying theory. It became one of the most influential and cited scientific books of the 20th century.
Towards the Alpha-Helix
In 1937, at the age of 36, Linus Pauling was named chairman of the Department of Chemistry and Chemical Engineering at Caltech, which had one of the most important chemistry programs in the world. Under his leadership, a great era in chemistry began at Caltech.
The arrival of the geneticist Thomas Hunt Morgan at Caltech in the late 1920s contributed to Pauling's growing interest in biological molecules. He began to participate in biology seminars; as Mary Jo Nye emphasised, “His reading in biology began to affect his thinking about chemistry, including his adoption of the term hybridisation to describe the 'changed quantisation' of the chemical bond.” By 1935, he had begun to speculate more generally about the properties of the large molecules found in living organisms. During the 1930s, his interest was focusing more and more on the new field of molecular biology, biochemistry, the study of enzymes and viruses, and research into blood molecules.
His early work in this area included investigations on magnetic properties of haemoglobin, in collaboration with Charles Coryell. He demonstrated that the haemoglobin molecule changes structure when it gains or loses an oxygen atom. Further studies on the denaturation process, in which the native configuration of a protein is altered, led him to emphasise the importance of the hydrogen bond for stabilising proteins. When these bonds are broken, the molecule assumes a more random configuration. The haemoglobin work further stimulated Pauling's interest in the structure and function of proteins. Proteins are involved in every major reaction in, and form an important part of every major structure of, the human body. Discovering how proteins were built and work, meant one step towards discovering the secret of life. At about that time, many people became interested in the problem of their three-dimensional structure. But some proteins consisted of tens of thousands of atoms joined into giant molecules, orders of magnitude larger than anything Pauling had studied before. It was proposed that all proteins were basically the same in the sense that they were all long chains of basic units, the amino acids, but differed in their specific properties especially because of their final shape. Discovering these shapes and understanding how they conferred on proteins their different amazing abilities became a major question. Pauling began looking for an answer and thought of building a model of proteins, starting from what was known of the structure of amino acids. In collaboration with his young assistant Robert Corey, he used X-ray crystallography and successfully discovered the structure of the simplest amino acid, glycine. The structure of a good number of amino acids and other peptides were later determined in Pauling's laboratory. In continuing to learn about these building blocks, Pauling also worked hard to understand more about the properties of whole proteins.
During World War II, Pauling dedicated himself to practical work. He discovered an artificial substitute for blood serum and invented an oxygen detector that found wide use in submarines and airplanes. He also studied explosives, rocket propellants and inks for secret writing. Near the end of the war, he learned that the red blood cells of patients with sickle-cell anaemia, a hereditary disease, become sickle-shaped only in venous blood, with low oxygen content. Three years later, Pauling and his collaborators were able to confirm that the sickling was indeed caused by a genetic mutation in the globin portion of the cell's haemoglobin, as he had hypothesised. Pauling himself called it history's first “molecular disease.”
Fascinated with antibody-antigen reactions, he developed a theory explaining how the antibody protein molecule could obtain a unique three-dimensional configuration that would provide it with specificity for a particular antigen. His research on the problem of the specificity of interaction of antibodies and antigens, led him to think that atoms and groups which form the surface of the antigen attract through weak intermolecular forces certain complementary parts of the globulin chain and repel other parts creating a unique folding of the antibody's polypeptide chain so that when its ends encountered an antigen molecule, they assumed configurations complementary to the surface regions of the antigen. This work with antibodies confirmed for him the powerful idea that much of the specific activity of biomolecules in the body could be explained through the close fitting of molecule to molecule, which he called “detailed molecular complementarity.” According to Pauling, complementarity was the core principle of molecular biology. This matching of shapes could explain phenomena from an enzyme's specificity for a certain substrate up to the greatest mystery in biology: how living organisms replicate themselves. By that time it was known that genes, small units of inheritance, carried the instructions for new generations, but their chemical nature was entirely unknown. Like most scientists, Pauling believed that genes were most likely made of proteins. But the mechanism through which genes make the precise copies of themselves needed to create new cells and new generations was completely unknown. The complementarity idea led him to hypothesise in 1948 that the use of a gene as a template would lead to the formation of a molecule with a complementary structure. He speculated that if the structure that serves as the template consists of two parts, which are themselves complementary in structure, then each of these could serve as the mould for the production of a replica of the other part, and the complex of two complementary parts could thus serve as the mould for the production of duplicates of itself. His description of the duplication process is incredibly similar to the DNA molecule, four years before its structure was discovered by Watson and Crick.
In 1948, Pauling returned to a problem that had occupied him in the late 1930s: the three-dimensional structure of proteins. Using what his laboratory had discovered about the structure of amino acids and the nature of the peptide bond, as well as his ideas about hydrogen bonds, Pauling set about building a mental model of alpha-keratin, a very common protein, the stuff of hair, fingernails and animal horn. But nothing seemed to work. A good deal of X-ray work had been done in England, at Lawrence Bragg's laboratory. British researchers thought that keratin and other proteins might have a spiral molecular structure. Shortly after learning this, during a sojourn in London, Pauling gathered paper, ruler and pencil, and began sketching out a chain of linked of amino acids, making the proportions as precise as he could, and marked the peptide bond with double-thick lines to indicate where the atoms were held rigidly in place. The key to the solution of the three-dimensional protein structure was to keep the peptide groups planar, with the same rotation angles in each peptide group. This put serious constraints on possible structures and much to his surprise actually led Pauling to a helical structure. By folding the paper to form a spiral, a structure came out that maintained his planar peptide bond, developing in a cylindrical coil-like configuration, later called the alpha-helix. But something was wrong and it was not possible to correlate the alpha-helix with the X-ray diffraction pattern of alpha-keratin. The discrepancy could not be removed by minor adjustments to the model and thus he published his findings only after three years, following an article by Lawrence Bragg, Max Perutz and Cowdery Kendrew, who were proposing potential helical structures for alpha-keratin, all unacceptable in Pauling's view. As Pauling later clarified: “I knew that if they could come up with all of the wrong helices, they would soon come up with the one right one, so I felt the need to publish it.”
Between February and March 1951, Pauling published a series of articles in the Proceedings of the National Academy of Sciences, some of which in collaboration with H. R. Branson and especially with his collaborator Robert Corey, a skilled X-ray crystallographer, in which he deduced the two main structural patterns of proteins: the alpha-helix and beta-sheet, now known to form the backbones of tens of thousands of proteins. The precise bond lengths and bond angles they had found from their past studies of crystal structures of amino acids and peptides, the components from which proteins are built up, imposed a series of constraints on structure that actually led Pauling to propose a helix in which the most important feature was that all six atoms of the amide (or peptide) group, which joins each amino acid residue to the next in the protein chain, lie in a single plane. The confirmation of the structure in this sense came from Max Perutz, who realised that that Pauling and Corey's alpha-helix was actually like a spiral staircase in which the amino acid residues formed the steps and the height of each step was 1.5 Angstrom. Perutz immediately observed in keratin the characteristic X-ray reflection of this regular repetition and also found the 1.5-Angstrom reflection in diffraction from haemoglobin, which he considered “the most thrilling discovery” of his life. Pauling's alpha-helix was found as well to be an important component of many other proteins of widely varying types. In 1962, Perutz and Kendrew would share the Nobel Prize in Chemistry “for their studies of the structures of globular proteins.” In another article, Pauling and Corey reported the discovery of a hydrogen-bonded layer configuration of polypeptide chains, in which the planar peptide groups lie in the plane of the sheet, and successive protein chains can run in opposite directions, giving an antiparallel sheet, as well as a parallel sheet. Beta-sheets were first seen in globular proteins, such as egg white lysozyme, in 1965.
The Double Helix of DNA: a Missed Discovery
Alpha-helix was an important structural feature of many proteins, but it seemed to offer no general explanations of protein activity. Pauling had not solved the mystery of life. Besides, it was becoming clear that the stuff that made genes were not proteins at all. From decisive set of experiments by the American microbiologists Alfred Hershey and Martha Chase, completed in early 1952, it became clear that genes were made of a long-chain molecule, a polynucleotide called deoxyribonucleic acid, or DNA. Hershey continued to work along this line and in 1969 he would share the Nobel Prize in Physiology or Medicine with Max Delbrück and Salvador Luria “for their discoveries concerning the replication mechanism and the genetic structure of viruses.” As soon as Pauling learned about these important experiments in the summer of 1952, he immediately switched his attention to DNA feeling that it should be possible to decipher the structure of this substance by building models along the lines of the successful protein models. In 1953, as scientists in several parts of the world were trying to determine its structure, Pauling and Corey published a paper in which they described DNA as a triple-stranded helix. The three strands were twisted around each other in rope-like fashion, with the phosphoric acid groups triangularly arranged in the centre and the various bases pointed outward. The analysis was unfortunately based on rather poor photographs published many years before and on equally poor photographs made in their laboratory.
A few months later Francis Crick and James Watson, who were part of Bragg's group at Cavendish Laboratory, published their famous paper describing the DNA molecule as a double helix. For their attack on DNA, they carefully followed Pauling's well-established techniques of model building and they, too, had considered a three-stranded structure a few months earlier, but had been lucky enough to show their attempt to Rosalind Franklin, Maurice Wilkins' assistant at nearby King's College and a skilled X-ray crystallographer, who had discovered that DNA came in two forms - a dry condensed form and a wet extended form. Other researchers worked with samples that were, unknown to them, mixtures of the two structures. The wet fibres made a simple cross shape, the characteristic sign of a helix, as Franklin knew. She had also deduced the location of the phosphate sugars known to be in DNA, but more pieces of the puzzle had still to be deciphered, even if she supposed that both forms of DNA were helical. She wanted more hard evidence before coming to a conclusion. Based on her work, she expressed complete disagreement with the three-strand model for the structure of DNA proposed by Watson and Crick.
Using a technique developed by Maurice Wilkins and adding several experimental refinements, she was later able to get excellent X-ray photos of the pure “wet” form of DNA. In spring 1953, through Maurice Wilkins, Watson and Crick were even more lucky to have access to Rosalind Franklin's most recent and especially clear DNA X-ray cruciform pattern, which clearly originated from a helix-shaped molecule, and to her results published in an internal report, without her knowledge or permission and before she had the chance to publish the photographs and her detailed analysis.
All this put them on the right track. Crick immediately drew the conclusion that the molecule should possess a twofold axis of symmetry, thus pointing to two chains running in opposite directions and definitely excluding a three-chain structure. They began feverishly devising models, now focusing on two-stranded models.
Within a few months, the two of them came up with a new DNA structure made of two chains wound around each other to form a spiral. Each strand was a complementary mirror image of the other, and each could act as a mould for forming a new double helix identical with the original. The “double helix” corresponded exactly to what Pauling had described as a likely feature of the genetic material four years earlier: each chain, when separated, could form another, identical chain. The Watson-Crick model immediately provided a mechanism for replication, which Pauling's model, with its bases facing out and unrelated to each other, could not. The discovery of DNA structure had a tremendous impact on molecular biology, setting the stage for new world-changing discoveries.
The following year Pauling was awarded the Nobel Prize in Chemistry “for his research in the nature of the chemical bond and its application to the structure of complex substances.” Since the prize was not for Pauling's more recent discoveries, the presentation speech could not refrain from remarking that Pauling's discoveries and his theories on structure had played a fundamental role for the interpretation of the complex structures of the large protein molecules and represented a fundamental contribution to the development of protein chemistry.
Rosalind Franklin died in 1958 of cancer, almost certainly due to the effects of the X-rays. She was thirty-seven years old. She had made crucial contributions to one of the most important discoveries of the twentieth century. Her later work on two other major biological problems and the techniques for solving them helped lay the foundations of structural molecular biology.
Watson and Crick shared the Nobel Prize in Physiology or Medicine 1962 with Maurice Wilkins, “for their discoveries concerning the molecular structure of nucleic acids and its significance for information transfer in living material.”
Bibliography
J. D. Dunitz (1996) Linus Carl Pauling, Biographical Memoirs of Fellows of the Royal Society 42: 316-338
Eisenberg D. (2003) The Discovery of the Alpha-Helix and Beta-Sheet, the Principal Structural Features of Proteins. Proceedings of the National Academy of Sciences 100 (20): 11207-11210
Goodstein J. R. (1984) Atoms, Molecules, and Linus Pauling. Social Research 51 (3): 691-708
Hager T. (1998) Linus Pauling and the chemistry of life, Oxford University Press
Hager T. (1995) Force of Nature. The life of Linus Pauling, Simon and Schuster, New York
Laylin K. J. Ed. (1994) Linus Carl Pauling. In Nobel Laureates in Chemistry 1901-1992, pp. 368-379
Nye, M. J. (2008) Pauling, Linus Carl.Complete Dictionary of Scientific Biography. Vol. 24. Detroit: Charles Scribner's Sons, pp. 36-44. Gale Virtual Reference Library
\url{http://go.galegroup.com/ps/i.do?id=GALE%7CCX2830905991&v=2.1&u=mpi_vb&it=r&p=GVRL&sw=w&asid=ae123bca88c1a010f3454be2eb7fb73e}
Pauling C. (1970) Daedalus 99 (4)The Making of Modern Science: Biographical Studies: 988-1014
Wasson, T. (ed.) (1987) Pauling Linus C. In Nobel Prize Winners, H. W. Wilson Company, New York, pp. 798-801