Prof. Dr. E. M. Mills Purcell > Research Profile
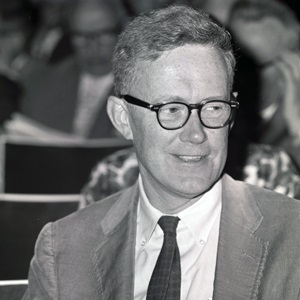
by Luisa Bonolis
E. M. Purcell
Nobel Prize in Physics 1952 together with Felix Bloch
"for their development of new methods for nuclear magnetic precision measurements and discoveries in connection therewith".
Edward Purcell is especially known in the physics community for his 1945 discovery with Henry Torrey and Robert Pound of a technique for measuring nuclear magnetic moments in bulk matter. For this discovery he was awarded the Nobel Prize for physics in 1952, jointly with Felix Bloch, who had independently and simultaneously investigated the same problem at Stanford.
Nuclear magnetic resonance, initially conceived as a way to reveal magnetic moments of atomic nuclei, led to an extraordinary range of developments and became a major technique for research in material sciences, chemistry, and of course medicine, where magnetic resonance imaging is now an indispensable tool.
Using nuclear magnetic resonance techniques, Purcell then found in 1951 with Harold Ewen that atomic hydrogen in the interstellar medium emits a radio-frequency signal at 1420 MHz. The giant clouds of hydrogen, unobservable by traditional optical methods, thus became visible at the 21-centimetre wavelength. Purcell and Ewen then designed and constructed the first radio telescope, which opened a new observational window for astronomical studies, allowing for the mapping of the overall structure of the galaxy despite the obscuring galactic dust clouds, in particular for the determination of the Galactic rotation curve. Since then, radio spectroscopy of atoms and molecules in space, through the detection of the hyperfine transition in hydrogen, has become a major part of the ever-expanding field of radio astronomy.
War Experience at MIT Radiation Laboratory
Edward Purcell was born in Taylorville, Illinois, and, after an education in public schools, he entered Purdue University in Lafayette, Indiana, in 1929. He received a B.S. in electrical engineering in 1933, but at that time his interests had already turned to physics. After spending a year at the Technical University in Karlsruhe, Germany, with an exchange fellowship, Purcell began graduate studies in physics at Harvard University, where he received his Ph.D. in 1938. During World War II, Purcell joined the Massachusetts Institute of Technology Radiation Laboratory, established for the purpose of developing microwave radar systems that would exploit the cavity magnetron, a revolutionary source of 10-cm electromagnetic waves that had just been invented by the British physicists John Randall and Henry Boot. During this important period of his scientific life, Purcell was associated with Isidor Rabi, who was the head of the Research Division and was already widely famous for his celebrated studies of molecular and atomic properties by means of radio waves. At MIT Rad Lab, Purcell supervised the Fundamental Studies Group, which was devising new techniques for the production and detection of microwaves. All this work involved technical problems never before encountered and new technologies to be developed, so Purcell's mind during the five years of war was on microwave radiation, its production, its transmission and detection. The work on the microwave radar was carried out against the backdrop of the Nazi threat. And after the 10-cm radar system was in production, physicists began to push the technology down to shorter wavelengths.
A major gain from these developments was the improved resolution, especially from aircraft, where space considerations seriously limited the antenna dimensions and therefore the sharpness of the beams. After an experimental 3-cm system was operating, going down to 1-cm was chosen as the next step. However, as the warmer weather of the spring of 1944 arrived, a disappointing decrease in the detection range was found. This was soon recognised to be associated with the increasing atmospheric humidity that brought with it a serious absorption of that particular wavelength by water molecules. Their choice had, unfortunately, put them right in the middle of a water vapour absorption band; hence, the range of the new system depended on the water content of the air. The urgent need for quantitative information of the absorptivity of air due to water vapour, which came to be known as the “water problem,” led to direct measurements of microwave absorption by a number of methods. Purcell's concern with the absorption of microwave energy by matter directly influenced his later approach to nuclear magnetic resonance.
Nuclear Magnetic Resonance With Molecular Beams
During his association with Rabi, Purcell had directly learned from him and his collaborators about the technique for measuring magnetic moments using molecular or atomic beams obtained by vaporising the sample to be studied. The fundamental experiments of Isaac Stern and his collaborators Otto Frisch and Immanuel Estermann, in which they determined in 1933 the magnetic moments of the proton and the deuteron by deflections of molecular beams in strong inhomogeneous fields, aroused Rabi's interest. He greatly developed this technique and carried out a series of brilliant investigations with his collaborators at Columbia University and measured the proton's as well as the deuteron's magnetic moment with increasing precision. In 1937, they had devised a new method in which the magnetic moments were directly determined by means of a system of different magnetic fields. Each beam particle was sequentially deflected in a first strong inhomogeneous field, and then in a second one, whose field strength was set to exactly undo what the first magnet did, so that all particles were again refocused in the detector. Between the two deflecting magnets there was an oscillating field superimposed at right angles to a strong, constant, homogeneous field.
The static magnetic field exerts a torque on the component of each magnetic moment that is not aligned along the field. The magnetic moment begins to precess around the field with a frequency called the Larmor precession frequency, proportional to the strength of the field through the gyromagnetic ratio, a constant characteristic of each nucleus or nucleon. The gyromagnetic ratio is also the constant of proportionality between the magnetic moment and the nuclear spin angular momentum. The strength of the constant magnetic field can be slowly varied, thereby varying the Larmor precession frequency and bringing it into coincidence with the frequency of the oscillating field, which can be controlled precisely by a radio-frequency oscillator. A free proton in a magnetic field will be found in one of two possible states, which differ in the orientation of the spin axis of the proton with respect to the magnetic field H. If the alternating field satisfies Bohr’s frequency condition for the energy difference between the two states, the resonance condition is achieved, producing a tilt of the nuclear moments. The transition occurring from one Zeeman level to another is analogous to the resonance absorption of visible light. However, instead of optical frequencies, one deals here normally with frequencies in the radio range, so that this application of the magnetic resonance method is properly labelled as belonging to the new field of radiofrequency spectroscopy.
Many atoms flip to another orientation and thus are no longer focused onto the detector by the second inhomogeneous field: The observed intensity diminishes registering a marked resonance minimum. If the spin is known, the simultaneous knowledge of the resonance field and the Larmor frequency then directly yields the gyromagnetic ratio and makes it possible to determine the nuclear magnetic moment with extraordinary precision. Knowledge of such properties characterising the fundamental constituents of the nucleus, the proton and the neutron, was of course very important to understand the behaviour of the nucleus on the whole.
The magnetic resonance method discovered late in 1937 led to the knowledge of the magnetic moments of the proton and the deuteron with an accuracy of about one part in a thousand. It was a great advance over previous molecular-beam methods for measuring magnetic moments. These measurements provided indications about the neutron, as well. If it was assumed that the deuteron was a compound nucleus made up of the proton and the neutron, and if, within the confines of the deuteron, the magnetic moments of the proton and deuteron were assumed to be additive, then the magnetic moment of the neutron could be inferred from a knowledge of the magnitudes and the signs of the proton’s and the deuteron’s magnetic moments, even if a direct measurement of such a quantity remained an open problem. During Purcell's stay at MIT Rad Lab, the 1944 Nobel Prize for Physics was awarded to Rabi for his pre-war research on molecular beams, where the concept of nuclear magnetic resonance had been applied for precise measurements of magnetic moments.
Without being aware of Rabi’s contemporary advancement of his research with molecular beams through the resonance method, during the summer 1937 Bloch had the idea of using a polarised beam of neutrons that would pass through a region of constant magnetic field which could be varied to the point where the Larmor precession of the neutrons was in resonance with the frequency of an oscillating magnetic field. The ratio of the resonance value of the constant field to the known frequency of the oscillating field of course could immediately give the value of the magnetic moment, according to the resonance condition. During 1939 Bloch and Luis Alvarez used the Berkeley 37-inch cyclotron to provide a sufficient flux of neutrons for the experiment. The beam of neutrons obtained by passing the unpolarised neutron beam from the cyclotron through the polariser, a strongly saturated plate of magnetised iron, emerged with a great number of neutrons oriented antiparallel to the magnetising field. Off resonance, the degree of polarisation remained intact. At the value of the resonance, the polarisation of the incident beam was changed, and the scattering of the beam in the second plate could be detected. Since the intensity of the neutron beam emerging from the analyser depended on the polarisation, the intensity was a function of the oscillating field frequency. At the observed value of the Larmor resonance the value of the magnetic moment could be directly determined.
In an article submitted to the Physical Review in October 1939, Alvarez and Bloch reported for the magnetic moment of the neutron a value of 1.935 ± 0.02 nuclear magnetons, with a sign negative with respect to the proton’s moment. This result was consistent with the magnetic moment of the deuteron measured by Rabi and collaborators, assuming the additivity of the proton and neutron moment. The war had interrupted these experiments, and Bloch was involved in radar research, working at Harvard Radio Research Laboratory. But when war was coming to an end, he began to think again about his neutron experiments and began to do some calculations. The experience with radio techniques later proved instrumental in inspiring his new work with nuclear magnetic resonance.
Purcell, on his side, not only learned a whole new kind of physics as well as research tools, especially microwave technology, but was also thrown together in a working relationship with a number of physicists from other places, in particular physicists from Rabi's laboratory at Columbia, from whom he learned enough about what they had done in molecular beams to begin thinking about what one could do in the way of resonance with what had been learned.
Nuclear Magnetism and Resonance Absorption in Solids
At the end of World War II, the MIT Radiation Laboratory prepared to close down, but several members, including Purcell, were asked to stay on and to also contribute to the writing of a series of books that would preserve the technology developed over five years at the laboratory and its collaborating organisations under conditions of military secrecy. In late summer 1945, Purcell was discussing about the possibility of detecting nuclear magnetic resonance as an absorption of radio-frequency energy with two friends and colleagues, Robert Pound and Henry Torrey, the latter being one of the Rabi laboratory veterans at Columbia, many of whom had actively worked in microwave developments during the war. On the one hand, Purcell had been led to think along these lines in writing about the absorption of microwaves by water vapour. The absorption was attributed to two energy states of the free H2O molecule that happened to have an energy difference corresponding to the quantum energy of the K-band radio waves. On the other hand, the knowledge acquired about resonance methods stimulated Purcell to bring up the idea of using a magnetic field to split the energy levels of a hydrogen nucleus and to use the frequency of a radio signal to measure the nuclear magnetic moment of a proton.
He proposed that they should jointly design and undertake an effort to detect resonant absorption of radio-frequency energy by atomic nuclei in solid matter held in a strong magnetic field. In order to understand the basic principle one can consider that the magnetic moments of protons contained in a small sample of water or paraffin wax are oriented in a completely random manner in the absence of an external magnetic field. When the sample is placed in a magnetic field, the moments immediately begin to precess with the Larmor frequency, regardless of their spatial orientation, and at the same time the component of the magnetic moment aligned with the magnetic field is pointing either parallel or anti-parallel. These two orientations form a two-level system: i.e., a system that has two states and a definite energy difference, or splitting, between them. Photons with the same energy as the splitting are at the resonance frequency with the system and can thus cause transitions between the states. The resonance frequency, in turn, depends on the strength of the static magnetic field and occurs in the radio-frequency region of the spectrum for a relatively strong magnetic field (about 7000 Gauss). A sample of protons put into a magnetic field is expected to have an equal population of parallel and anti-parallel moments. Radio waves at the resonance frequency would then be expected to have an equal chance of causing energy absorbing transitions - from a lower to a higher state - and energy emitting transitions. After a certain time, called the relaxation time, the sample of protons will attain thermal equilibrium with respect to the magnetic field. At equilibrium, there will be a slight difference in population between the two orientations, because more protons will be in the state of lower-energy, i.e., of parallel orientation. A radio signal at the resonance frequency will cause more upward transitions and the detected signal is thus proportional to the population difference between the states so that, in net, absorption will be observed. NMR is a rather sensitive spectroscopy since it is capable of detecting these very small population differences. It is the resonance, or exchange of energy at a specific frequency between the spins and the spectrometer, which gives NMR its sensitivity.
Torrey was initially sceptical, for he thought that the difference in population between the two energy levels would be so small that the energy absorbed from a normal radio signal would be minuscule. But after some calculations he found that the signal could perhaps be detected after all.
Using inactive Radiation Laboratory equipment, Purcell, Torrey and Robert improvised an experiment at Harvard Research Laboratory of Physics, where they had the possibility of using a magnet, built in the 1930s by Curry Street to bend the tracks of cosmic rays in a cloud chamber placed in the gap between the poles of the magnet. In 1936, with this magnet Street had been able to discover a track that was actually due to a new particle, which later was called muon. This magnet was strong enough to sufficiently split the energy levels. On December 15, 1945, after some unsuccessful attempts, Purcell and his collaborators succeeded in detecting the absorption of radio waves by protons in paraffin wax by magnetic resonance.
The essence of their magnetic resonance experiment was to detect what they described as a transition between two quantum energy states, which took place when the hydrogen nuclei absorbed energy and were excited to the higher Zeeman level.
The heart of their system was an electromagnetic cavity oscillator whose inductive part was filled with solid paraffin, the substance to be investigated, rich in hydrogen, and thus in protons. The cavity was placed between the poles of the large cosmic-ray magnet, which supplied a static magnetic field. The resonant cavity itself was part of a bridge circuit, which was inductively coupled to a receiver and especially sensitive to small changes in signal intensity,. The circuit was driven with an rf-signal generator at a fixed frequency, and the radiofrequency magnetic field in the cavity was everywhere perpendicular to the steady field. The cavity output was balanced in phase and amplitude against another portion of the signal generator output. With the rf circuit balanced the strong magnetic field was slowly varied, thereby changing the energy separation between the two Zeeman energy levels. At resonance, the energy-level separation and the fixed frequency of the weak oscillating magnetic field satisfied the Bohr relation for the quantum leap between the two states. The protons absorbed energy and caused a change in the balance of the radiofrequency bridge and an extremely sharp resonance absorption could be observed.
Later experiments, which involved the development of more sensitive instrumentation for nuclear magnetic resonance studies, involved the graduate student Nicolaas Bloembergen, just arrived from Utrecht. The team of Bloembergen, Purcell and Pound carried out a series of fundamental experiments in 1946 and 1947. The report on this work, published in 1948 in the Physical Review, established a record for its citations. Bloembergen's later activity in the field on NMR culminated in the proposal for a three-level solid-state maser in 1956, when the laser era was in its early stage. In 1981 Bloembergen was awarded the Nobel Prize for Physics jointly with Arthur Leonard Schawlow, “for their contribution to the development of laser spectroscopy.”
Gerstein reported Purcell's later comments on the experiment, in which he describes it as a novel synthesis of sure principles: “The ingredients are the law of physics, well-established things: the proton has a magnetic moment; I put on a magnetic field at the right frequency and it absorbs. Each one of these, by that time, was a well-established law of physics. If I put them all together, and if I haven't forgotten anything, it has got to do what the laws tell it to do... If it had not worked, that would have been a real discovery!”
Bloch, too, had returned to Stanford from the Harvard Radio Research Laboratory with clear ideas about the experiments he wanted to perform. The essential fact of the magnetic resonance consists in the change of orientation of nuclear moments, and the methods to be employed in molecular and atomic beams as well as in neutron beams were primarily dictated by the different ways of detecting this change. The acquaintance with radio techniques during the war suggested to Bloch still another and much simpler way, that of detecting the electromagnetic induction caused by the flip in the orientation of nuclear moments through the normal methods of radio reception. A coil carrying an alternating current and producing an oscillating magnetic field in the x direction will induce at resonance a reorientation of the magnetic moment. Bloch thought that, therefore, one could expect an oscillating induced voltage in a receiver coil, oriented with its symmetry axis along the y direction. It was for this reason that he considered the term nuclear induction most suitable and descriptive of the phenomenon as a whole. The exact value of the frequency that gives the maximum signal can then be used to calculate the magnetic moment. With his collaborators William Hansen and Martin Packard, Bloch prepared a set of coils surrounding a spherically shaped container of water. The axes of the coils were oriented at right angles to each other and the whole system was placed between the poles of a magnet that produced a static magnetic field in a fixed direction.
In December of 1945, Bloch and Purcell met at the annual meeting of the American Physical Society and realised that they were working on similar problems. They decided that Bloch would continue his researches and investigate liquids, whereas Purcell would concentrate on crystals and solids in general. Their results appeared at the same time and both methods came to be known as nuclear magnetic resonance (NMR), soon to become the foundation of new fields in physics, chemistry, biology, physiology and medicine. As emphasised by Rigden, Bloch and Purcell were very familiar with Rabi's work, and Bloch had also independently arrived at the idea of resonance. However, they conceptualised the resonance method in bulk matter in different ways. For Bloch, “the observation of the resonance point is based upon the fact that in its neighbourhood there will be a finite probability P for a change in orientation...” For Purcell, on the other hand, “In the well-known magnetic resonance method for the determination of nuclear magnetic moments by molecular beams, transitions are induced between energy levels...” Of course they were referring to the same phenomenon and actually the two methods, resonance absorption and nuclear induction, are essentially equivalent, even if Bloch's method permits the signs of magnetic moments to be determined.
The method of Bloch and Purcell implied a great simplification and generalisation in this respect, enabling its application to solid, liquid and gaseous substances. Since each kind of atom and its isotopes have a sharply defined and characteristic nuclear frequency, one can for any object placed between the poles of an electromagnet seek out and examine with radio waves all the various kinds of atoms and isotopes present in the object in question. The advantage is that this can be done even in only small quantities, and, most importantly, without in any perceptible way affecting the sample, its form, crystalline structure, etc. An analysis in situ of extraordinary sensitiveness is thus obtained, which can be adapted to many scientific and technical fields. This was truly a cutting edge technology. For this achievement Bloch and Purcell were jointly awarded the 1952 Nobel Prize for Physics.
Since then the field of nuclear magnetism has enormously grown in importance, both for its basic scientific interest and surprising technological applications. In particular, the nuclear magnetic resonance spectrometer has become a standard tool for any laboratory concerned with analytical chemistry, completely usurping the role of the Bunsen burner in earlier days. Resolution nuclear magnetic resonance spectroscopy became one of the most rapidly expanding branches of chemistry. It is thus not surprising that, starting from 1965, a new dedicated journal, Progress in Nuclear Magnetic Resonance Spectroscopy, began to be published in order to make available the continuous supply of up-to-date authoritative reviews dealing with such topics.
The practical value of magnetic resonance methods has been immense also in biology. The magnetic resonance imaging method, or MRI, represented the greatest advance in medical imaging since the discovery of X-rays in 1895. It can be viewed as complementing X-ray studies, since it allows investigations into physiological and metabolic processes.
Bibliography
Bleaney B. (1999) Edward Mills Purcell. 30 August 1912-7 March 1997. Biographical Memoirs of Fellows of the Royal Society 45: 438-447
Gerstein M. (1994). Purcell’s role in the discovery of nuclear magnetic resonance: Contingency versus inevitability. American Journal of Physics 62: 596-601
Pound R. V. (2000) Edward Mills Purcell. 1912-1997. Biographical Memoirs of the National Academy Press 78: 3-24
Ramsay N. R. (1999) Early history of magnetic resonance. Physics in Perspective 1: 123-135
Rigden J. S. (1986) Quantum states and precession: The two discoveries of NMR. Reviews of Modern Physics 58(2): 433-448
Wasson, T. (ed.) (1987) Purcell, Edward M.. In Nobel Prize Winners, H. W. Wilson Company, New York, pp. 841-843