Prof. Dr. Melvin Schwartz > Research Profile
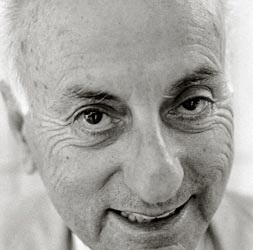
by Roberto Lalli
Melvin Schwartz (1932-2006)
Nobel Prize in Physics 1988
Schwartz’s Formative Years Between New Particle Accelerators and New Detectors
Melvin Schwartz was born in the Bronx, New York City, on 2 November 1932. Soon after World War II, Melvin Schwartz attended the eminent Bronx High School of Science, which, established in 1938, was becoming one of the premier public schools for secondary education in the natural sciences. Schwartz’s interest in physics began as soon as he entered this school at the age of 12. In 1949, Melvin Schwartz enrolled at the Physics Department of the Columbia University, where Schwartz took his bachelor's degree in 1953. At the time, the Columbia physics department headed by I. I. Rabi was a highly stimulating environment for physicists and physics students. Rabi had been building a research group in which the interconnections between experimentalists and theorists were exceptionally fruitful. In addition, the growth of economic support for physics in the aftermath of World War II was allowing for the construction of new facilities devoted to high-energy physics. Columbia researchers could work at the Columbia Nevis Laboratory, which in the late 1940s became home of the most powerful synchrotron of the world. Moreover, the close Brookhaven National Laboratory (BNL) was formally established in 1947, with the primary purpose to serve the universities of the East Coast. In this lively environment, Schwartz pursued his PhD studies in experimental physics, encountering many of the physicists with whom he would collaborate, including the Chinese-American theoretical physicist T. D. Lee, and the experimental physicist L. Lederman, as well as J. Steinberger, who was Schwartz’s thesis advisor.
During his PhD, Schwartz was part of the team of researchers working under J. Steinberger on the first bubble chamber experiments at the BNL. The bubble chamber was invented in 1952 by D. Glaser. It was rapidly understood that bubble chambers could be made larger, and could detect more energetic particles, than the cloud chambers. For this discovery, Glaser was awarded the Physics Nobel Prize in 1960.
In 1953, the proton synchrotron of the BNL—the Cosmotron—reached its maximum energy of 3.3. GeV, becoming the first accelerator to operate in the GeV range. The Columbia group in which Schwartz was working developed the techniques and the instruments for employing the bubble chamber at the Cosmotron. This research project led to several relevant discoveries in the 1950s, including the demonstration of parity nonconservation in hyperon decay (1957), the discovery of the neutral Σ0 hyperon (1957), and the discovery of the beta decay of pions (1958)—i.e., the discovery that pions could decay into electrons, not only into muons. After earning his PhD in 1958, Schwartz began his career within the faculty of Columbia University, where he stayed for the next 17 years.
Putting the Elusive Neutrino to Test
After physicists had reached a certain degree of consensus about the quantum mechanical description of atomic physics, they tried to extend the range of application of the theory to the nuclear domain. Many of the properties of the nuclei, however, were difficult to understand on the basis of the accepted theoretical framework. Some eminent physicists also wondered whether, within the nucleus, one had to give up the conservation principles. One of the most disturbing features of nuclear behaviour concerned the continuous spectrum of the emission of electrons (the beta decay). Such a spectrum seemed to imply that the conservation of energy and momentum did not hold in beta decays. In order to save the conservation principles, in 1930 Pauli hypothesised the existence of a massless (or nearly massless), uncharged and spin ½ particle. Pauli supposed that this particle was produced in beta decays allowing for the conservation of the aforementioned quantities. Fermi christened this hypothetical particle ‘neutrino’ and, in 1933, put forward a quantum field theory for beta decays based on the emission of electrons and (anti)neutrinos.
The success of Fermi’s theory made the neutrino a plausible entity although it was extremely elusive from the experimental point of view. The neutrino interacted only weakly with matter. According to Fermi’s theory, an interaction between neutrinos and other weakly interacting particles occurred extremely rarely. For instance, calculations predicted that the depth necessary to make one neutrino interact with lead atoms was a light year of lead.
In spite of these discomforting predictions, theoretical constructs concerning weak interactions took for granted that neutrinos actually existed, and substantial efforts to provide an experimental proof of their existence began in the early 1950s. Frederick Reines hypothesized that neutrinos might be produced in sufficient quantity in the vicinity of nuclear weapon explosions. Soon after, Reines and Clyde Cowan realized that the same held for nuclear reactors, because of the elevated flux of anti-neutrinos produced in the decay of fission fragments. Such neutrinos could interact with protons, leading to the inverse beta decay: ν + p → n + e+. In June 1956, Reines sent an enthusiastic letter to Pauli: The hypothetical particle Pauli had proposed 26 years earlier had finally been detected. Besides the fundamental discovery of the neutrino, the Cowan-Reines experiment was also important because it clarified some fundamental aspects of the weak interactions. The absence of any reaction of the form ν + n → p + e- proved that the lepton number was conserved (by convention electrons and neutrinos have lepton number L = 1, while positrons and anti-neutrinos have lepton number L = -1). Despite the importance of the discovery, the achievement was regarded worthy of the Nobel Prize only some 30 years later, when Reines was awarded half of the 1995 Nobel Prize in Physics “for the detection of the neutrino.” In his Nobel lecture, Reines remembered his close collaborator Cowan who had had passed away in 1974 and could not share the Prize with him.
The Discovery of the Muon-Type Neutrino
In the aftermath of World War II, particle physics was in the midst of a process of rapid, and somewhat confused, transformation, both theoretical and experimental. The number of new particles being discovered and the difficulties in providing a theoretical framework for describing their properties was at its peak. As for the weak interactions, observational data and their theoretical interpretations led to the understanding that there existed different particles with similar features. In the 1940s, it was established that the electron was not unique: The µ-particle (or muon) behaved in a similar way, but was about 200 times heavier than the electron. In the 1950s, studies on the decays of the pions and the muons demonstrated that all the neutrinos related to these decays had the same helicity as the neutrinos emitted in beta decays. The most common interpretation of this empirical feature was that neutrinos (and anti-neutrinos) appearing in the weak interactions were the same particles, whatever the lepton involved.
In 1958, in order to solve some theoretical incongruences of the universal V-A theory of weak interactions, G. Feinberg suggested that instead there might be different neutrinos; more specifically, that the muon (anti-muon) and electron (positron) both had their own anti-neutrino (neutrino). This supposition became known as the two-neutrino hypothesis. Rabi’s efforts to construct a working environment in which theoreticians and experimentalists worked in close contact proved to be fundamental for the development of the research on high-energy neutrino beams and the discovery of the muon neutrino. During the usual coffee hour of discussion, in 1959, the Chinese-American theoretical physicist T. D. Lee discussed the possibility of producing high-energy neutrino beams in laboratory accelerators. Many discussants had strong objections to Lee’s suggestions and the discussion ended with the general impression that such a setup was not feasible.
Schwartz, however, developed Lee’s idea and, in a few months, proposed a methodology for the production of beams of high-energy neutrinos as well as for their detection. In a one-page paper published in 1960 under the title “Feasibility of Using High-Energy Neutrinos to Study the Weak Interactions,” Schwartz proposed that pion decay might serve as the source of an effective high-energy neutrino beam. (Pontecorvo was in the same period developing a series of theoretical considerations that led to the same conclusions). A high-energy, high-intensity proton beam impinging on a solid target produced a huge number of charged pions (fig.1). The high-energy pions rapidly decay into muons (anti-muons) and anti-neutrinos (neutrinos).
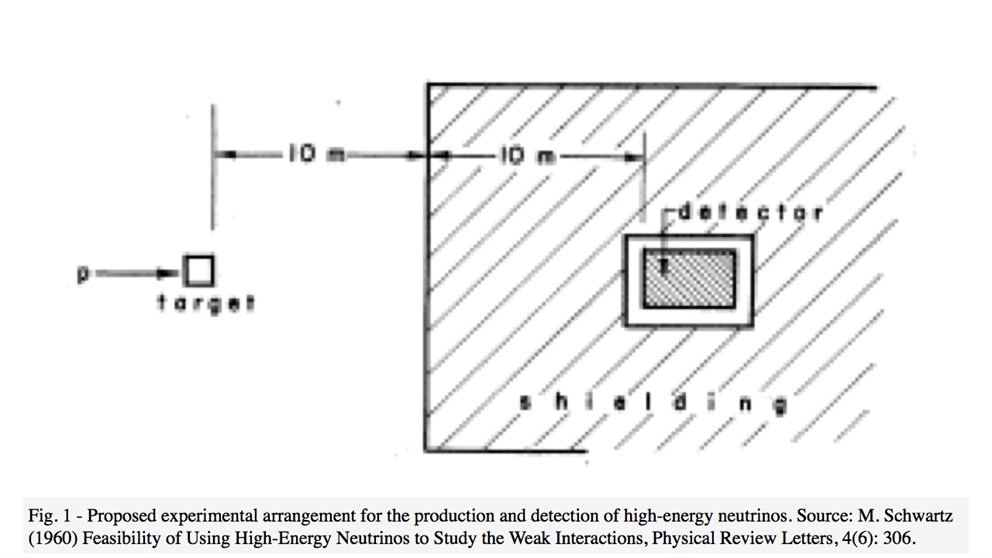
Subsequently, a massive shield stopped both pions and muons, while the neutrinos (anti-neutrinos) remained mostly untouched. The neutrinos had relatively high energy and could be observed by a detector, which Schwartz left undefined. Although the accelerators then employed were not powerful enough to produce an observable high-energy neutrino beam, Schwartz was optimistic that the more powerful accelerators then under construction might be used.
In the meantime, C. N. Yang and Lee claimed that the existence of two different kinds of neutrinos was essential to explain the absence of the decay µ → e + γ. Experimental researchers of the Columbia team began collaborating to design an experiment that could establish whether the neutrino appearing in the pion decay was different from the one of the beta decay. The concept of the experiment was rather simple. If all the particles produced by the detector could be identified as muons, it would have meant that neutrinos produced in pion decays were different from the beta-decay ones and, thus, that there existed, at least, two families of leptons. If both electrons and muons were produced, the experiment would have instead shown there existed only one type of neutrino.
On May 1960, a Columbia team composed by Lederman, Schwartz, Steinberger, and others submitted a proposal to the BNL to perform such an experiment at the newly-constructed Alternating Gradient Synchrotron (AGS), which with its 33 GeV of maximum energy, reached in June 1960, had become the world’s premier accelerator. A recent advancement in detecting techniques proved to be very useful for the group’s goal. The recently developed spark chamber allowed for the counting of singular events in which electrons and muons were more clearly distinguishable than in bubble chambers. After a trip to Princeton to study the spark chamber of the Cronin group, Schwartz began constructing the spark chamber to be employed in the experiments for the observation of the neutrino-related interactions. After many days of observations at the AGS, the experimenters became confident of having isolated about 30 events that might be interpreted as muons, produced by high-energy neutrino interactions in the spark chamber. No electron was observed. In the description of their result the experimenters were rather sure about the interpretation of their data: “[t]he most plausible explanation for the absence of the electron showers […] is then that vµ ≠ ve; that is, that there are at least two types of neutrinos.” The experimentalists also summarised the various theoretical arguments that made the two-neutrino hypothesis attractive. Their reasoning convinced the majority of physicists that leptons were divided in at least two families of particles. This discovery was fundamental in the development of the theory of weak interaction that would later be part of the Standard Model of particle physics. The experiment also proved that high-energy neutrino beams could be effectively produced and, therefore, it pioneered the introduction of a novel experimental methodology for the empirical study of weak interactions, which would later be relevant also for the understanding of the properties of quarks within the nucleons. In 1988, when the Standard Model was already the mainstream framework for the understanding of particle physics, Melvin Schwartz, Leon Lederman and Jack Steinberger were awarded the Nobel Prize in Physics "for the neutrino beam method and the demonstration of the doublet structure of the leptons through the discovery of the muon neutrino."
A Life Between Pure and Applied Science
After 17 years at Columbia, in 1966 Schwartz accepted a position as group leader at the Stanford Linear Accelerator (SLAC), which was then beginning operations. There, he investigated the charge asymmetry in the decay of long-lived neutral kaons. Schwartz was also involved in a project aimed at producing and detecting relativistic hydrogen-like atoms made up of a pion and a muon. He conducted the latter experiment at the BNL and at the Fermilab, which was established in 1967. Schwartz’s simultaneous activity at the three most important HEP facilities of the United States made him the suitable person for strengthening the communications links between the various research projects.
Annoyed by the growth of bureaucracy in the HEP world, in the 1970s, Melvin Schwartz founded, with the SLAC engineer Leon Birkwood, Digital Pathways—a company specialised in computer security. In 1983, Schwartz resigned from his position at the SLAC to dedicate his full time to the firm of which he was also president until 1990. In 1991, Melvin Schwartz decided to come back to pure physics research as Associate Director of High Energy and Nuclear Physics at the BNL. His work on experimental high-energy physics was again limited by economical constraints due to the enormous growth of the funds needed for performing new experiments. In 1994, Schwartz went back to his Alma mater where he was nominated I. I. Rabi Professor of Physics from 1994 to 2000, when he retired as Rabi Professor Emeritus.
Bibliography
Fitch V. L., & Rosner L. (1995) Elementary Particle Physics in the Second Half of the Twentieth Century. In Brown, L., Pippard, B., & Pais, A. (Eds.). Twentieth century physics (Vol. 2). AIP, New York, pp. 635-794.
Hoddeson, L. Brown, L. M., Riordan, M. & Dresden, M., (1995) The rise of the Standard Model: Particle Physics in the 1960s and the 1970s. Cambridge University Press, Cambridge.
Pais, A. (1986) Inward Bound Of Matter And Forces In The Physical World. Clarendon Press, Oxford.
Pickering, A. (1999) Constructing quarks: A sociological history of particle physics. University of Chicago Press, Chicago.
Reines, F. (1996) The Neutrino: From Poltergeist to Particle. Reviews of Modern Physics, 68(2), pp. 317-327.
Samios N. P., & Yamin P. (2012) Melvin Schwartz 1932-2006. Biographical Memoir of the National Academy of Sciences.
http://www.nasonline.org/publications/biographical-memoirs/memoir-pdfs/schwartz-melvin.pdf
Schwartz, M. (1989) The First High-Energy Neutrino Experiment. Reviews of Modern Physics, 61(3), pp. 527-532.