Prof. Dr. Frederick Soddy > Research Profile
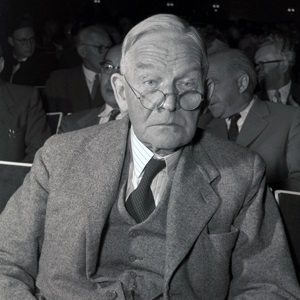
by Luisa Bonolis
Frederick Soddy
(1877-1956)
Nobel Prize in Chemistry 1921
“For his contributions to our knowledge of the chemistry of radioactive substances, and his investigations into the origin and nature of isotopes”.
The “Chemistry of Phantoms”. The Soddy-Rutherford Collaboration
Frederick Soddy, the son of Benjamin Soddy, a London merchant, was born at Eastbourne, Sussex, England, on September 2, 1877. After being educated at Eastbourne College, where his science master R. E. Hughes encouraged him to study chemistry, he obtained a scholarship to Merton College, Oxford. In November 1895, when Soddy was beginning his studies at Oxford University, Wilhelm Conrad Röntgen was carrying on his experiments on a new kind of rays of unknown nature, capable of photographing invisible things like the bones of a living hand and of exciting fluorescence in a paper plate coated with barium platino-cyanide. In the wave of excitement following the discovery of X-rays, as Röntgen had named the mysterious radiation, the French physicist Henri Becquerel, an expert in phosphorescence and fluorescence, thought that phosphorescent materials, such as some uranium salts, might emit penetrating X-ray-like radiation when illuminated by bright sunlight. By chance he found that uranium salts spontaneously emitted some radiation able to produce an image on a photographic plate without being exposed to the sun. By Spring 1896 he had become convinced that the penetrating radiation came from uranium itself. What he had discovered was radioactivity, a term later coined by Pierre and Marie Curie, who used it to describe the constant emission of radiation by uranium minerals. Their research led to the discovery of additional radioactive elements: thorium, polonium and in particular radium, which they named after the Latin radius, meaning ray, because its radiation is 3 millions times greater than that of uranium.
In 1901, Röntgen was the first physicist to be awarded the Nobel Prize, while the Curies were duly awarded the 1903 Nobel Prize in Physics for studies into “radiation phenomena”, along with Becquerel for his discovery of “spontaneous radioactivity”. In 1911, Marie Curie would also be awarded the Nobel prize in Chemistry for her part in the discovery of radium and polonium.
In clearly stating that the spontaneous and continuous emission of radiation by radioactive elements was a specific “atomic” process, the Curies were setting the stage for the new science of radioactivity, in which Frederick Soddy would be soon involved.
In the meantime, Soddy had graduated in 1898 with First Class Honours in Chemistry, with Sir William Ramsay as external examiner. After being engaged in independent chemical research at Oxford for two years, he moved to Canada in Spring of 1900, accepting a position as demonstrator at the Chemistry Department of McGill University in Montreal. There he met the brilliant physicist Ernest Rutherford, who had taken up the chair of Experimental Physics at McGill University in the autumn of 1898. The Physics Building, donated by the tobacco millionaire William Macdonald, was reputed to be one of the finest and best equipped in the world. Rutherford had arrived in Cambridge from New Zealand in 1894, at the time of Röntgen’s discovery, and had started to work as a graduate student under J. J. Thomson at Cavendish Laboratory. In 1897, Thomson had performed the well known experiments on the magnetic and electric deflection of cathode rays which demonstrated the existence of the electron. For his investigations on the conduction of the electricity by gases, Thomson was awarded the Nobel Prize in Physics 1906.
Rutherford joined Thomson in these researches, but in the meantime great events had happened in France. The discovery of new radioactive elements had started a frenetic race to find more. Actinium was soon unearthed and many other substances were isolated from uranium and thorium so that Rutherford's interest switched to this brand new field. He began to investigate the ionization produced by uranium. In 1899 Rutherford established that the passage of what were still called “Becquerel rays” through foils revealed the existence of at least two quite separate types of radiation. One was easily absorbed, while the other had a greater penetrating ability. Rutherford named them alpha- and beta-rays “for simplicity”, but observed that “The cause and origin of the radiation continuously emitted by uranium and its salts still remain a mystery.” A yet more penetrating type of radiation was clearly described by Paul Villard in 1900 and called gamma-rays.
In 1900, when Soddy arrived at Montreal, Rutherford had just discovered that thorium - like uranium - constantly produced a material “emanation”, a radioactive gas, whose chemical nature at first eluded him. The puzzle increased when similar “emanations” were found associated with radium and actinium. The work of chemically separating the products of decay from the original source involved a great deal of careful chemistry, so that Rutherford soon recognized that he needed expert chemical assistance and involved Soddy in the study of “emanations”.
Rutherford was a very skilled experimental physicist, and Soddy was a chemist with a good grasp of atomic conceptions. They approached their experimental work from different directions, directions which turned out to be perfectly complemented in a winning strategy for studying phenomena connected with radioactive change. They were both very young - Rutherford was 29 and Soddy only 23 - and their collaboration lasted only eighteen months, from mid-October 1901 to mid-April 1903, but was one of the most interesting and fruitful in the history of science.
They provided experimental evidence that the constant production of a material emanation from thorium -which they eventually identified as a member of the noble gas group now known as radon - was produced not by thorium directly, but by some unknown, and apparently chemically different, element which they termed “thorium X”. Four days after the extraction, they found that the thorium X had lost half of its activity, while the original thorium had regained exactly as much activity as the thorium X had lost. This suggested that one element, thorium, can decay into a second element, thorium X, which in turn can lose its activity. The creation of new thorium X balanced the loss of activity. This was the first indication that radioactive substances were characterized by a new parameter, a life-time or a half-life.
They had now a firm case for natural transmutation: thorium must be regenerating the thorium-X, which later was actually discovered to be radium-234, an isotope of radium. One substance was produced as the result of the uncontrollable disintegration of another. The rate of the process was found in every case to be as the exponential law: in a fixed time interval, a fixed fraction of nuclei will decay. Based on this evidence, Rutherford and Soddy put forward the disintegration theory of radioactive decay in “The cause and nature of radioactivity”, published in two parts in 1902.
A refined form of their disintegration theory appeared in 1903, according to which a radioactive substance transformed into another substance in the sense that the atoms changed from a “parent element” to another “daughter element” at a characteristic rate. Not only do atoms change or transmute, they also do so randomly, such as expressed by the decay constant, that depends only on the nature of the radioactive element. In their 1903 paper, Rutherford and Soddy stated the general law of radioactive change, noting that transformation is represented by the expulsion of a charged particle, during which the chemical atom undergoes a disintegration.
As did other prominent workers in the subject, Rutherford and Soddy claimed that radioactivity was an atomic property, independent of physical surroundings or chemical composition, and stated that “The normal or constant radioactivity possessed by thorium is an equilibrium value, where the rate of increase of radioactivity due to the production of fresh active material is balanced by the rate of decay of radioactivity of that already formed.''
As Soddy himself recalled in his Nobel Lecture: “This was a theory sufficiently challenging to the accepted doctrines of chemistry.” Prior to the Soddy-Rutherford research, the common notion was that radioactive materials give off some form of energy, without undergoing any fundamental change themselves. Soddy was stunned by the colossal impact of the evidence they were finding: “Rutherford, this is transmutation: the thorium is disintegrating and transmuting itself into an argon gas.” Rutherford shot back: “For Mike's sake Soddy don't call it transmutation - they'll have our heads off as alchemists.” In print, they used the term “spontaneous transformation.” At the time, Rutherford and Soddy were in fact members of a very small circle of people working in this esoteric field, which by the 1920s widely came to be known in the popular press as the “modern alchemy” of atomic science, a tiny patch of ground sitting between physics and chemistry.
The close collaboration between Rutherford and Soddy, which established the laws of the spontaneous transmutation of the elements, also laid much of the foundations of subsequent developments in nuclear physics. It came to an end in 1903, when Soddy left Montreal to join Ramsay at University College in London to examine more fully the gaseous product of the decay of thorium. Ramsay, who had discovered helium in 1895, had also found the noble gases neon, krypton and xenon, guided by theoretical considerations founded on Mendeleev's periodic system. In July 1903, Ramsay and Soddy were able to see the typical spectroscopic yellow line of helium after the passage of sparks through the gas, experimentally confirming Rutherford and Soddy's suspicion that the decay of radium continuously produced helium. The following year Ramsay was awarded the Nobel Prize in Chemistry “in recognition of his services in the discovery of the inert gaseous elements in air, and his determination of their place in the periodic system.”
In 1908 Rutherford definitely clarified the long suspected identity of the helium so produced, with the expelled alpha-particles, and in December of that same year he received in Stockholm the Nobel Prize in chemistry “for his investigations into the disintegration of the elements, and the chemistry of radioactive substances.” Somewhat surprised that his work was regarded as Chemistry, he jokingly remarked during the Nobel banquet that he had “dealt with many different transformations with various periods of time, but the quickest that I have met was my own transformation in one moment from a physicist to a chemist.”
He chose as the subject for his Nobel Lecture “The Chemical Nature of the Alpha Particles from Radioactive Substances.” The very special nature of Rutherford's beloved alpha-particle actually represented a bridge between physics and chemistry, which would soon lead Rutherford to his nuclear model of the atom.
Isotopes, the Multiple Personality of Chemical Elements
In 1904, Soddy accepted a position as Lecturer in Physical Chemistry in Glasgow University, where he worked out in detail the chemical behaviour of the radioactive elements and developed a new, far-reaching conception related to radioactive elements. After his helium work in London, a further great contribution to the establishment of the disintegration theory was carried out in Glasgow: the proof of the growth of radium from uranium.
However, during the ten-year period following his 1904 appointment to the University of Glasgow, Soddy's attention was especially devoted to the chemistry of the radio-elements and in that period he developed two important conceptions which helped to clarify the relation between the increasing number of radio-elements resulting from the chain of decays and the periodic table. The first was the identity of chemical properties among some radio-elements, which came to be described as isotopes, and the second was the Displacement Law, the movement through the periodic table as a radio-active element emitted an alpha- or beta-ray.
The analysis of the constituents of matter which up to that time had been regarded as the most fundamental, was an analysis by means of the chemical property of elements. Although the powerful method of spectroscopic analysis had filled a few vacant places in the Periodic Law with some new elements, these were classified by chemists along with the rest of what were considered to be the building blocks of the material universe. The new method of radioactive analysis was applicable of course only to the radio-elements, such as uranium and thorium and the successive unstable products of their spontaneous disintegration, but at the time very few radiochemists existed in the world, all of them working in close contact with physicists. The transitory nature of the radio-elements, coupled with the exceedingly small quantities commonly handled, made many a traditional chemist hesitant to accept these unusual substances as real elements worthy of insertion into the periodic table. And indeed, in his presidential address to the chemistry section of the British Association in 1907, Arthur Smithell called work in radioactivity the ‘chemistry of phantoms’.
A plethora of substances growing from the different decay series had been discovered, but how all these elements could be fitted int the few remaining spaces in the periodic table was entirely unclear. Moreover, attempts to use basic chemistry to separate them from radioactive material were meeting with failure. Examples of radio-elements with extremely similar chemical properties accumulated, and in many cases two substances, which were known to have different radioactive properties and molecular masses, simply could not be separated from one another by chemical methods and appeared completely identical in their whole chemical character. By mid-December 1910, Soddy succeeded in establishing that mesothorium, just discovered by Otto Hahn, was in fact chemically identical to radium (it is actually radium-228). This recognition led Soddy to speculate that there were different forms of the same chemical element with identical chemical properties, but different atomic weights. During the annual report on the progress of chemistry for the year 1910, he admirably summed up the situation. As Otto Hahn - the future discoverer of fission and a skilled radiochemist himself - later remarked, this was an important step, because Soddy had the courage to declare that: “The conclusion is scarcely to be resisted that we have in these examples no mere chemical analogues, but chemical identities… [emphasis added].” Soddy also put forward the idea that such a property seemed destined to have its most important application in the region of the inactive elements, thus extending the concept to the whole table of elements. A. S. Russell and R. Rossi later demonstrated that the chemically indistinguishable products of the transformation of the radioactive elements were also spectroscopically identical.
By 1913, it was clear that the occurrence of isotopes was the rule rather than the exception. Forty distinct radioactive substances had been distributed over only ten slots of the periodic table: “it would not be surprising if the elements… were mixtures of several homogeneous elements of similar but not completely identical atomic weights,” claimed Soddy. At this time he was confident of this interpretation and used the term isotope - whose etymological meaning comes from ancient Greek isos (equal, same) and tópos (place) - to describe the various types of each element, belonging to the same position in the periodic table, but physically distinguishable because each had a distinct mass and half life. It was not the atomic weight of an element, but its nuclear charge which determined its place in the periodic table.
At that time, Henry G. J. Moseley, a pioneer in the use of the method of X-ray spectroscopy, was discovering that a systematic mathematical relationship exists between the wavelengths of the X-rays produced and the atomic numbers of the metals that were used as the targets in X-ray tubes. What has became known as Moseley's law, showed that the atomic numbers of elements are not just rather arbitrary numbers based on chemistry and the intuition of chemists, but rather have a firm experimental basis in the physics of their X-ray spectra. In addition, Moseley showed that there were gaps in the atomic number sequence at numbers 43, 61, 72, and 75. These spaces are now known, respectively, to be the places of the radioactive synthetic elements technetium and promethium and of the last two rare naturally-occurring stable elements hafnium (discovered by George de Hevesy in 1923) and rhenium (discovered in 1925).
Moseley's methods and analyses thus made the huge step of placing the concept of atomic number on a firm foundation based in physics.
In 1913, Soddy also showed that an atom changes into a new element by moving two places lower in atomic number upon alpha-emission. At about the same time, A. S. Russel and Kasimir Fajans, working independently, extended that law to include beta-decay, in which an element's atomic number increases by one after the loss of a beta-particle. The group displacement law, which is now known as the radioactive displacement law of Fajans and Soddy, was a fundamental step toward understanding the relationships among families of radioactive elements. This enabled the decay series to be plotted on a figure of mass against atomic number.
The novelty of the idea of isotopes was later shown to have important implications well outside of the field of radioactive elements where it was first recognized, also thanks to Francis W. Aston's systematic work performed at Cavendish Laboratory in Cambridge. Employing his mass spectrograph Aston was able to identify 212 naturally occurring isotopes, thus definitely validating Frederick Soddy's theory of isotopes.
Frederick Soddy was awarded the Nobel Prize in Chemistry for 1921 “for his contributions to our knowledge of the chemistry of radioactive substances, and his investigations into the origin and nature of isotopes.”
He received the prize in 1922, together with Francis W. Aston, who won the 1922 Nobel Prize in Chemistry “for his discovery, by means of his mass spectrograph, of isotopes, in a large number of non-radioactive elements, and for his enunciation of the whole-number rule.”
Bibliography
Alexander Fleck (1957). Frederick Soddy. Born Eastbourne 2 September 1877 Died Brighton 26 September 1956. Biographical Memoirs of Fellows of the Royal Society 3: 203-216
Thaddeus J Trenn (1981). Soddy, Frederick. Complete Dictionary of Scientific Biography. Vol. 12. Detroit: Charles Scribner's Sons: 504-509
Thaddeus J Trenn (1977). The self-splitting atom: The history of the Rutherford-Soddy collaboration, London: Taylor & Francis
Freeman M. I. (1979). Soddy, Frederick and the Practical Significance of Radioactive Matter. British Journal for the History of Science 12 (42): 257-260
Oswald U. Anders (1964).The place of isotopes in the periodic table: The 50th anniversary of the Fajans-Soddy displacement laws, Journal of Chemical Education, 1964, 41 (10): 522-525
George B. Kauffman (1986). Frederick Soddy (1877-1956): Early Pioneer in Radiochemistry (Chemists and Chemistry). D. Reidel Pub. Co.