Prof. Dr. Jack Steinberger > Research Profile
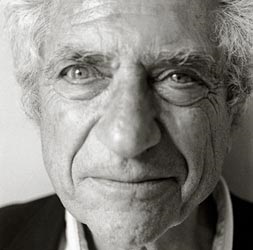
by Roberto Lalli
Jack Steinberger
Nobel Prize in Physics 1988
On the Border Between Theoretical and Experimental Physics
Hans Jakob "Jack" Steinberger was born on 25 May 1921, to Jewish parents in Bad Kissingen (Bavaria, Germany). After the Nazi party came to power in 1933, Steinberger’s parents decided to send him to the USA in order to escape the anti-Semitic politics in Germany. Supported by a US businessman, Steinberger pursued scientific studies in Chicago. His education was broad, ranging from engineering—which he studied at the Armour Institute of Technology (now Illinois Institute of Technology) for two years—to chemistry, in which he majored at the University of Chicago in 1942.
On 8 December 1941, the USA entered World War II, and Steinberger’s scientific preparation made him suitable for joining one of the several US research activities related to the war efforts. At the MIT Radiation Laboratory (Rad Lab), Steinberger was offered his first formal training in physics. He became acquainted with classical electromagnetic theory and received an introduction to quantum mechanics and solid-state physics.
After the end of the war, Steinberger decided to pursue PhD studies in physics and obtained an assistantship at the University of Chicago. During World War II, physicists had been massively employed in US military activities. The two atomic bombs dropped on Hiroshima and Nagasaki in August 1945 had dramatically demonstrated that physicists would be essential for the military strength of nations in the future. Everywhere—and especially in the USA—physics enjoyed a period of rapid evolution thanks to the number of new academic and research positions as well as to an enormous increase in government funds for research endeavours. Enrico Fermi was one of the leaders in US nuclear research, and his name attracted many students to the University of Chicago, including C. N. Yang, T. D. Lee, M. L. Goldberger, and O. Chamberlain.
In this stimulating environment, Steinberger began making active research in physics, with the intent of becoming a theorist. In his Nobel autobiography, Steinberger quoted four teachers that had the deepest influence on him: E. Teller, W. Zachariasen, G. Wentzel, and Fermi. The style of the latter, in particular, strongly impressed Steinberger. In a period of increasing specialization—when theoretical and experimental physics had become sharply separated from each other—Fermi continued to be considered a leading expert in both subdisciplines. Fermi’s attempt to build strong connections between theoretical and experimental physics would become crucial in Steinberger’s future switch from theoretical to experimental physics.
Steinberger’s peculiar position on the border between experimentation and theory was already evident in his efforts to find a topic for his dissertation. He wanted to make theoretical research on subatomic particles. When Steinberger began his PhD, the number of known subatomic particles was relatively small with respect to the particle zoo that would shape physics in the 1950s. Physicists were particularly concerned with a particle discovered by C. Anderson and S. Neddermeyer in 1936 through cosmic ray observations, which was then usually referred to as the mesotron (now muon). In 1947, an empirical discovery helped clarify the properties of the mesotron. A team composed of C. Lattes, G. Occhialini, C. Powell, and others discovered that there were two kinds of particles of similar mass, but very different lifetimes. The π-mesons (pions) interacted strongly with matter and could rapidly decay in µ-mesons (namely, the mesotrons), which interacted only weakly. After the discovery of the pion, µ-meson became the official name of the mesotron.
It was known that negatively (positively) charged µ-mesons decayed in electrons (positrons) and it was supposed that this decay also produced the hypothetical neutrino in analogy with the Fermi theory of nuclear beta decay: µ- => e- + ν; or µ+ => e+ + ν.
Steinberger interpreted the data of an experiment on the stopping of slow µ-mesons recently performed by Rossi, Sands, and Sard, as an indication that the energy of the outgoing electrons was less than expected on the basis of the two-particle picture, i.e., the hypothesis that the µ-meson decayed into only two particles.
Fermi encouragged Steinberger to test his idea experimentally, and this eventually became the topic of his dissertation. At the end of 1948, Steinberger concluded his measurements of the energy spectrum of the decay electrons; they strongly suggested that the µ-meson decayed to one electron and two neutrinos; i.e., µ => e + ν + ν. In 1948, Steinberger earned his PhD and published the result of his experiment in the paper “On the Range of the Electrons of Meson Decay.” Steinberger’s results, along with previous experiments on µ-meson capture and lifetime, led other theoreticians to propose the so-called Universal Fermi Theory of Weak Interaction, according to which beta decays, µ-meson captures and µ-meson decays were different aspects of the same interaction based on a single coupling constant.
After his PhD, Steinberger went for a postdoctoral period at the Institute for Advanced Study in Princeton, which, under the direction of Oppenheimer, was becoming the mecca of theoretical physics. In 1948, a group of postdoctoral students led by F. Dyson was busy trying to digest the new renormalization techniques that had just been developed by S.-I. Tomonaga, J. Schwinger, R. Feynman, and Dyson himself.
Steinberger struggled to find a topic on which he could make original research and was not very productive in this period. Only at the end of his stay at the Institute, he made a publishable theoretical analysis. Steinberger estimated the decay rate of both π-mesons and µ-mesons employing regularization methods. Steinberger’s calculation of the lifetime of the neutral pion decaying in two photons was particularly important. The neutral pion, first postulated in 1937 by N. Kemmer, had not been observed yet. Steinberger calculated that the neutral pion lifetime could be much shorter than the experimental upper bound measured up to then.
After his year at the Institute for Advanced Study, Steinberger’s career as a theoretical physicist continued only for a few months as assistant of the Italian theoretical physicist G. C. Wick at the University of California at Berkeley. The possibility of employing the powerful particle accelerators that were under construction at the Berkeley Radiation Laboratory provided a strong stimulus for Steinberger to switch to experimental physics. Steinberger had at his disposal the 330 MeV electron synchrotron built under the direction of Edwin McMillan to perform experimental tests on the neutral pion. Steinberger developed a counting technique to detect pions, which was employed in a series of experiments performed with various co-workers. These experiments confirmed the existence of the neutral pion and provided a measure of its lifetime at 2.62x10-8 sec—a value very close to the one accepted today.
From this moment onward, Steinberger did not change his field of research any more, becoming one of the leaders of experimental physics in a period marked by the discoveries of an enormous number of new particles as well as by the transition from cosmic ray observations to laboratory accelerators. Steinberger’s career, however, had to continue in different sites. Since Steinberger refuted to sign the so-called “Non-Communist Oath” of the University of California, he was not re-appointed and, in 1950, he accepted a position at Columbia University, where he would stay for the next 18 years.
New Accelerators, New Detectors, New Particles
Between the late 1940s and the early 1950s, Columbia University was at the forefront of experimental research in particle physics. Columbia University operated the recently established Nevis Laboratory, which by the late 1940s housed the most powerful cyclotron of the world. In addition, the close Brookhaven National Laboratory (BNL) was opened in 1947 and was became one of the largest facilities for particle physics experiments. Steinberger continued his research on the pions, employing the 385 MeV cyclotron of the Nevis Laboratory. The research Steinberger made with several collaborators allowed for a clarification of the properties of the pions. In 1954, a group headed by Steinberger employed for the first time the recently constructed liquid-hydrogen bubble chamber as a detector in particle accelerators. The team, which included the PhD student M. Schwartz, performed their experiments with the Cosmotron—a proton synchrotron that in 1953 had reached its maximum power, accelerating protons to 3.3. GeV. The combination of the enormous energy reached by the Cosmotron and the reliability of the new detection technique of the bubble chamber allowed for several discoveries concerning the strange particles and their properties. In 1954, Steinberger’s group demonstrated the existence of the Sigma hyperon and measured its mass. In the following years, Steinberger headed research leading to the demonstration of parity violation in hyperon decays (1957)—a few months after parity violation had been detected for the first time—the demonstration that the pions also decayed into electrons (1958), and the determination of the parity of the neutral pion (1962).
Hunting for the Neutrino(s)
The neutrino is perhaps the most elusive particle. Pauli first hypothesised its existence in 1930 to explain the continuity of the beta decay spectrum. After the Fermi theory of beta decay (1933) began to be considered as the right theoretical framework to understand the emission of electrons by nuclei, the neutrino hypothesis gained consensus within the physics community. Theoreticians continued to employ neutrinos as essential elements, although these particles were not detected until 1956. The enormous difficulty in observing neutrinos is due the extremely small probability that these almost massless and uncharged particles interact with matter. To overcome this difficulty, F. Reines thought to employ nuclear explosions as sources because of the enormous quantity of neutrinos liberated during this process. Reines and Cowan later developed this intuition and decided to detect neutrinos produced by a recently built nuclear reactor.
Neutrinos interact only weakly with matter. It was, thus, necessary to consider the inverse of the beta reaction in order to calculate the outcome of a neutrino (or, more correctly, anti-neutrino) interaction; i.e., ν+ p => n + e+. This reaction could be detected because the positron rapidly annihilates with an electron, producing a pair of photons, and the neutron also releases a photon when captured by a nucleus. The detection of the three gamma rays was soon understood as the signature that the inverse of the beta decay had occurred.
Since the late 1950s, it was believed that there was a unique neutrino appearing in all the weak interactions; in particular, the same kind of neutrino was supposed to appear in both muon and beta decays, as well as in muon capture. At the end of the 1950s, theoretical considerations led G. Feinberg to suggest that two different neutrinos existed—one related to the electron, the other to the muon. In 1960, Lee and Yang made an extended discussion of the possible result of hypothetical high-energy neutrino experiments. In particular, they claimed that the existence of two different neutrinos was in fact necessary to explain the absence of the decay µ = e + γ, which had been never observed.
The possibility to test the two-neutrino hypothesis was related to the problem of performing experiments on high-energy neutrinos. This was a very subtle problem, and many physicists doubted that it was possible to create a source of high-energy neutrinos. The Columbia researcher M. Schwartz and, independently, B. Pontecorvo conceived an experiment that could in principle produce a beam of high-intensity high-energy neutrinos. The idea was to employ a beam of high-energy protons accelerated against a target. The outcome of the interaction protons–target would be a beam of high-energy pions that rapidly decay in muons and the related neutrinos (or anti-neutrinos). Schwartz also discussed the feasibility of the proposed experiment and argued that it would be soon realizable, thanks to the novel accelerator then under construction at the BNL.
A Columbia team including Steinberger, Leon Lederman, Schwartz and others began elaborating on Schwartz’s original idea to build the first high-energy neutrino beam. In May 1960, the team submitted a proposal to the BNL to perform such an experiment at the Alternating Gradient Synchrotron (AGS), employing a bubble chamber as detector. The AGS was completed in July 1960, when it reached the energy of 33 GeV—then the highest energy produced by particle accelerators. While the group was completing the design of the apparatus, in September 1960, they decided to replace the bubble chamber with the recently invented spark chamber. The spark chamber was much more effective for the type of experiment the group had in mind because it allowed for a clear distinction between electrons and muons. The final design was much more complex than the one initially proposed by Schwartz because it had to take into account all the possible background effects (fig1).
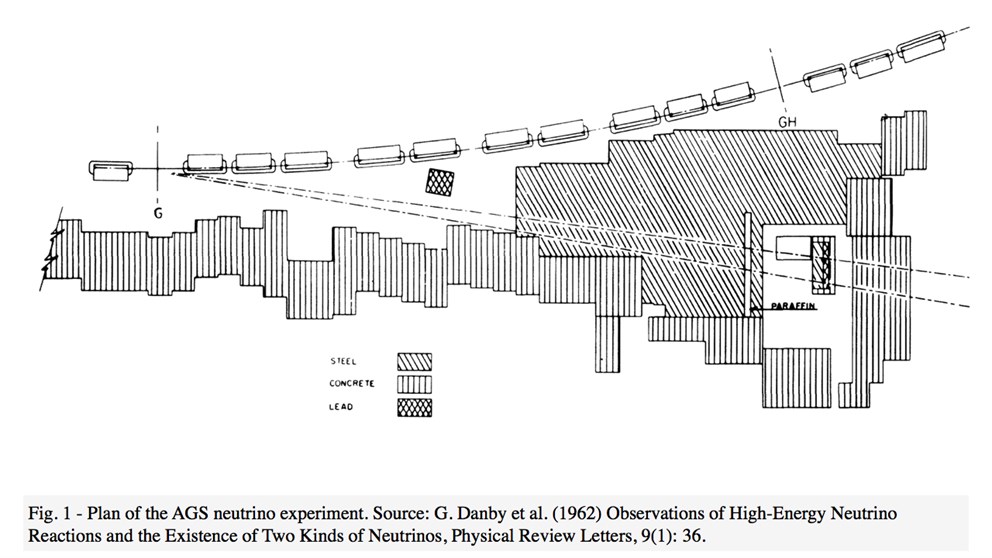
The experiment was directed towards the study of the interactions induced by high-energy neutrinos. Following the theoretical analysis made by Yang and Lee, the Columbia experimental team was particularly interested in providing evidence that could corroborate, or confute, the two-neutrino hypothesis.
The reasoning went as follows. The main decay products of high-energy charged pions were charged muons and the related neutrinos, according to the reaction π => µ + ν. The high-energy neutrinos produced by this reaction later interacted weakly with matter, producing leptons. In case, the two-neutrino hypothesis was correct the neutrinos liberated by the reaction could interact with matter only in reactions such as: ν + n => µ- + p; or ν+ p => µ+ + n.
The two-neutrino hypothesis implied that the only leptons produced by the final interaction of high-energy neutrinos were muons. If the outgoing particles detected by the spark chamber were only muons, it would have meant that there existed two different types of neutrino. In case both electrons and muons were produced, the experiment would have shown that there existed only one type of neutrino.
On June 15, 1962, the group of researchers submitted their result to Physical Review Letters, rapidly published with the title “Observations of High-Energy Neutrino Reactions and the Existence of Two Kinds of Neutrinos.” The experiment, they claimed, offered clear evidence that only muons were produced by the high-energy neutrinos interactions. As a consequence, the two-neutrino hypothesis gained momentum and was rapidly accepted by the physics community. A second noteworthy outcome of this groundbreaking experiment was that, from then onward, neutrino beams could be employed to study the properties of weak interactions. In 1988, Steinberger, Lederman, and Schwartz were awarded the Nobel Prize in Physics “for the neutrino beam method and the demonstration of the doublet structure of the leptons through the discovery of the muon neutrino.”
Leading Experimental Physics Between Two Continents
After the momentous discovery concerning the different families of leptons, Steinberger continued to do research at the BNL till 1968, when he left to join CERN as department director. There, Steinberger began employing a new detector of charged particles and photons that had been invented by G. Charpak in 1968: the multiwire proportional chamber (MWPC). The MWPC decisively improved the efficiency of observation by increasing from ca. 1 to ca. 1000 the number of particles that could be detected in a second. Moreover, it was possible to analyse the electronic signal of the particles with computers. “For his invention and development of particle detectors, in particular the multiwire proportional chamber“ Charpak was awarded the Nobel Prize in Physics in 1992.
Between 1968 and 1995, Steinberger headed projects that employed high-energy neutrino beams for the study of particle interactions. These projects were able to determine more accurately the Weinberg angle of electroweak interactions, demonstrated the existence of right-handed neutral currents, and, also, provided some empirical supports to Quantum Chromodynamics, the theory of strong interactions (QCD). Lastly, Steinberger was the spokesperson of a group of 350 physicists who collaborated in the construction of the detector ALEPH at the electron-positron collider of the CERN (LEP). ALEPH began operating in 1989, providing, in the following years, strong confirmations of both the unified theory of electro-weak interactions and QCD. Steinberger continued to contribute to the research of the CERN both as a manager and a researcher until 1995, when he felt that he was no more able to work in a context, which was radically different, compared with the year in which he made his first experiment, by himself.
Bibliography
Fitch V. L., & Rosner L. (1995) Elementary Particle Physics in the Second Half of the Twentieth Century. In Brown, L., Pippard, B., & Pais, A. (Eds.). Twentieth century physics (Vol. 2). AIP, New York, pp. 635-794.
Hoddeson, L. Brown, L. M., Riordan, M. & Dresden, M., (1995) The rise of the Standard Model: Particle Physics in the 1960s and the 1970s. Cambridge University Press, Cambridge.
Pais, A. (1986) Inward Bound Of Matter And Forces In The Physical World. Clarendon Press, Oxford.
Steinberger, J. (1989) Experiments with high-energy neutrino beams. Science, 245(4923), pp. 1202-1208.
Steinberger, J. (1997) Early particles. Annual Review of Nuclear and Particle Science 47(1), pp. xiii-xlii.
Steinberger, J. (2005) – Steinberger Jack-Biographical. Nobelprize.org. Nobel Media AB 2013. (Accessed April 2014). http://www.nobelprize.org/nobel_prizes/physics/laureates/1988/steinberger-bio.html