Prof. Dr. Harold Clayton Urey > Research Profile
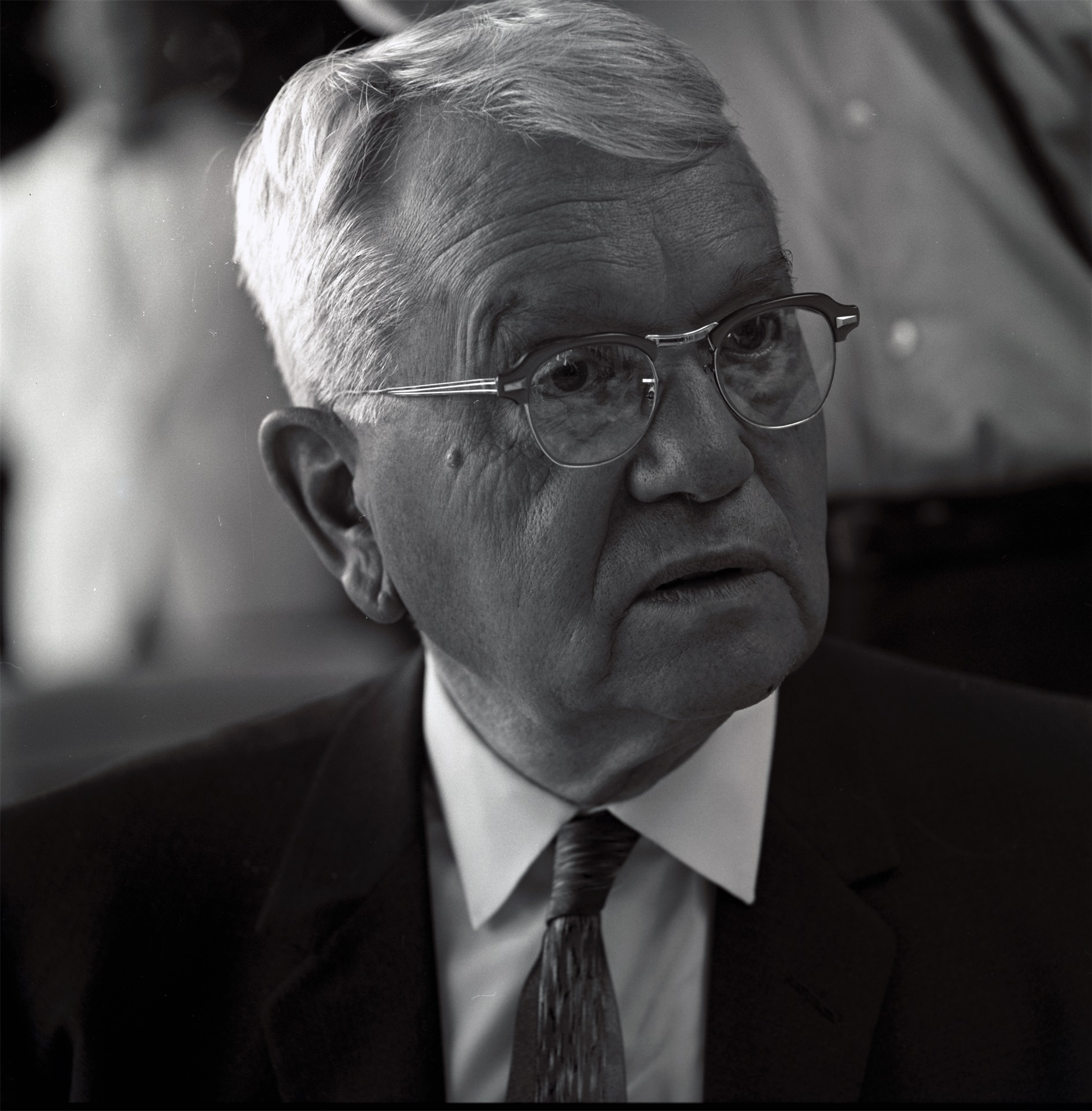
by Luisa Bonolis
Harold Clayton Urey
Nobel Prize in Chemistry 1934
“for his discovery of heavy hydrogen”.
Between Chemistry and Physics
Harold Clayton Urey was born in Walkerton, a small town of Indiana, in 1893. After graduating from high school in 1911, he taught for the next three years in rural schools and then decided to attend college and entered the University of Montana in Missoula. In 1917 he received a degree in zoology with a minor in chemistry and after working in a chemical industry on war materials, in 1921 he accepted a fellowship at the University of California, Berkeley for graduate work in chemistry with a minor in physics. The renowned chemical thermodynamicist Gilbert N. Lewis was Urey's nominal research director, but he actually chose his own line of research in thermodynamics, studying the rotational contributions to heat capacities and entropy of molecules consisting of two atoms. As a graduate student, Urey was a pioneer in the calculation of thermodynamic properties from spectroscopic data. He was fascinated by the new atomic physics and believed that the future of physical chemistry was quantum chemistry. After receiving his Ph.D. from Berkeley in 1923, he went off for a year at Niels Bohr's Institute for Theoretical Physics in Copenhagen with an American-Scandinavian Foundation fellowship. During this period Urey became involved in the international development of atomic and molecular physical science, and made the acquaintance of prominent scientists of the time, including Werner Heisenberg, Wolfgang Pauli, Hans Kramers and Georg de Hevesy. Also in Europe Urey met Albert Einstein, who became a life-long friend.
After returning to the U.S. in 1924 he served as Associate in Chemistry at Johns Hopkins and then moved to Columbia, where he was appointed Associate Professor of Chemistry in 1929. He was convinced that no real boundary existed between chemistry and physics, and that progress in chemistry depended on the application of quantum mechanics. In his research work of this period, he himself dealt with quantum mechanics and applications of molecular spectroscopy to chemistry, as well as other issues of primary interest to physicists. In 1930 Urey wrote Atoms, Molecules, and Quanta with the physicist Arthur Ruark, which emphasized the relevance of quantum theory to molecular structure and spectroscopy. Being the first comprehensive text on atomic structure written in English, it became the standard American text on chemical quantum theory for some years, firmly establishing Urey's professional reputation.
Hunting for the Heavy Isotope of Hydrogen
In 1930 Urey became interested in isotopes. The deep understanding that he had acquired of thermodynamics and quantum mechanics, together with his interest in the regularities of nuclear structure and his skills in optical spectroscopy led him to get interested one day of 1931 in a communication in The Physical Review written by Raymond T. Birge and Donald H. Menzel. The atomic weight of hydrogen as measured by chemical methods differed slightly from the physical value found in Aston's mass spectrograph. To reconcile this discrepancy, the authors were postulating the existence of a stable isotope of hydrogen of mass 2 present in ordinary water at 1 part in 4500 of the light variety.
The atomic weight of hydrogen had been a sore point of contention among scientists for a long time. Its value had led Rutherford to propose, in his famous Bakerian Lecture of 1920, not only his hypothetical neutron as a combination of a proton with a “nuclear electron,” but also one or more isotopes of hydrogen. The theory of isotopes - i.e., the idea that an individual element may consist of atoms with the same number of protons but with different masses - had been proposed independently by Frederick Soddy, in England and by Kasimir Fajans in Germany, in 1913. In 1913, Sir J.J. Thomson succeeded in separating isotopes of neon with atomic weights of 20 and 22, respectively, by passing a beam of neon ions through a magnetic field, which deflects an ion in proportion to the ratio of its electric charge and mass. This was the first experimental proof of Soddy's hypothesis that different atoms might occupy the same place in the Periodic Table. That same year, Arthur B. Lamb and Richard Edwin Lee, working at New York University, had reported a very precise measurement of the density of pure water, concluding that pure water does not possess a unique density. It was the first reported experiment in which an isotopic difference in properties was clearly in evidence. Speculations about isotopy gave rise to the building of mass spectrometers, employing electromagnetic focusing, capable of separating the isotopes of chemical elements. After World War I, improvements of J. J. Thomson's instrument allowed Francis W. Aston to identify more than two hundred of naturally occurring isotopes. In 1922 Aston received the Nobel Prize in Chemistry for his outstanding work which gave an extensive knowledge of the isotopes and their exact masses, and led to his formulation of the whole number rule which states that the mass of the oxygen isotope being defined as 16, all the other isotopes have masses that are very nearly whole numbers.
In 1931, when Urey read Birge and Menzel's paper, isotope research was in full bloom and almost all of the stable isotopes of light atoms that are known today had been found, mostly via mass spectroscopy. Researchers had accumulated much empirical knowledge on the nature of isotopes. The masses of isotopes had all been found to be close to integer multiples of the mass of atomic hydrogen, and the charges of all ions had been revealed to be multiples of the elementary charge of the hydrogen nucleus. However, the very existence of isotopes, their number, relative abundances and masses, were all questions to which answers were being sought. Urey and others constructed charts of the known isotopes to show relationships bearing on their existence. The reason for atoms of the same element having different weights was, however, unclear until the discovery of the neutron by James Chadwick in 1932, and the charts were based on the orthodoxy then still prevailing that atomic nuclei were composed of protons, plotted as ordinates, and “nuclear electrons,”plotted as abscissae. A reasonably accurate description of the masses of the isotopes came in fact from supposing that their nuclei consisted entirely of protons plus some “nuclear electrons” as needed to balance the nuclear charge. Their number was the number of protons minus the atomic number of the element. Nuclear electrons, which were also advocated to explain the emission of beta-rays by radioactive nuclei, actually implied a series of paradoxes from the point of view of quantum mechanics. They were banished from nuclei only by Fermi's theory of beta-decay of 1933.
The proposed hydrogen isotope of mass 2 (in units of the proton mass) which would arguably be the simplest example of an isotope, was just what was needed in order to fill in a prominent blank spot in the pattern of a systematic diagram of light isotopes that Urey had posted on his office wall. The proton-electron plot of known atomic nuclei showed marked empirical regularities among atoms of lower atomic number and up to oxygen-16 a simple step-wise figure appeared into which the nuclear species hydrogen-2 and hydrogen-3 could actually be fitted very nicely. Within days of reading the Birge and Menzel's article, Urey devised an experiment to look for hydrogen-2. As Urey wrote in his Nobel Prize lecture, he realized that “Bohr’s theory, given some 20 years ago, permits the calculation of the Balmer spectrum of the heavier isotopes of hydrogen from [the] spectrum of hydrogen.” However, the effect would be quite small, also because of the low natural abundance of the heavy isotope: one atom for every 4,500 atoms of hydrogen-1. Teaming up with his colleague George Murphy at Columbia, Urey made a first trial with gas from a commercial tank of hydrogen. They soon identified hydrogen and its isotope spectroscopically observing the Balmer atomic spectrum with a spectrograph designed with Murphy.
They could have stopped work son that first day, and write out a paper announcing the discovery of heavy hydrogen. However, to make really sure that the new spectral lines were not due to any external effect caused by the grating of the spectrograph, Urey decided to try to make samples of hydrogen in which the concentration of heavy isotopes would be enriched. Thanks to his mastery of thermodynamics, he calculated the low temperature vapour pressures of the isotopes and was able to determine that on distilling liquid hydrogen, hydrogen-1 atoms would evaporate faster, leaving behind a liquid in which heavier isotopes are concentrated, since heavier molecules have lower speeds of thermal motion, and should be less likely to evaporate from the liquid. To pursue this approach, Urey enlisted the help of Ferdinand G. Brickwedde, chief of the Low Temperature Laboratory of the National Bureau of Standards in Washington, D.C., who had already developed a reputation in low-temperature physics, even being only 28 years old. The samples were sent by railway express to Columbia University, where their emission spectra were recorded with Urey's high-resolution spectrometer, designed with his colleague George Murphy. At the wavelength that Bohr's theory predicted for the mass 2 isotope of hydrogen, there was a line that showed a four- fivefold increase in intensity of the Balmer lines of the hydrogen-2, according to the expected concentration of the sample. There was no evidence for an isotope of mass 3 which also had a blank spot in the chart of isotopes.
This discovery, which stemmed from Urey's deep understanding of thermodynamics, and quantum mechanics, together with his interest in the regularities of nuclear structure and his skills in optical spectroscopy was published in The Physical Review on New Year's Day, 1932. Just seven weeks later, James Chadwick announced the “possible existence of a neutron,” a neutral particle with a mass very nearly equal to that of the proton. From that time on, the isotopes of an element were viewed not as inseparable bodies, but as consisting of atoms which have the same nuclear charge (number of protons) but different masses (number of neutrons).
The naming of the new isotope involved a protracted debate involving mainly Urey, Gilbert N. Lewis, Ernest O. Lawrence and Ernest Rutherford. The problem was whether to retain the name ‘hydrogen’ for the new isotope, because, as sustained by Bohr it was not a new element, or give it a special name, because it was expected to be quite different chemically from ordinary hydrogen because of its double mass. Urey and his crew ultimately decided to name the hydrogen trio as protium, deuterium, and tritium, and published these names in the Journal of Chemical Physics on 1 July 1933.
But hydrogen-3, in spite of his brand new name, was still missing. Moreover, there was one question of particular interest to Rutherford and his colleagues at the Cavendish, and to Ernest O. Lawrence and Charles C. Lauritzen at Berkeley and Pasadena: namely, the use of hydrogen-2 nuclei as swift projectiles for the transmutation of elements. Carrying no more repelling charge than protons, but having twice their mass, they might well prove twice as effective. Transmutation of elements had already been obtained in 1932 - the annus mirabilis of nuclear and particle physics - by John D. Cockcroft and Ernest T. S. Walton at Cavendish Laboratory. They had found that lithium bombarded with fast protons from their new kind of accelerator - from then on known as the Cockcroft-Walton accelerator - was transformed in two helium atoms with the emission of swift alpha-particles. For “their pioneer work on the transmutation of atomic nuclei by artificially accelerated atomic particles” Cockcroft and Walton got the 1951 Nobel Prize in Physics.
Then, in March 1934, a Letter to Nature announced a new “sensational atom-smashing experiment” made by Lord Rutherford, M. L. Oliphant and P. Harteck in Cavendish Laboratory bombarding deuterium compounds with ions of heavy hydrogen. Two distinct reactions were apparently involved: in one, the target and projectile deuterons fused to produce tritium, the missing isotope of hydrogen consisting of one proton and two neutrons, which is a highly unstable isotope. In the second deuterium-deuterium reaction, the two deuterons fused to produce a helium-3 nucleus (two protons and one neutron), which is stable, but very rare in nature. In parallel with the discovery of a new heavy isotope of hydrogen, they had actually obtained the first man-made fusion reaction, opening a long and difficult struggle - still going on nowadays - in which both deuterium and tritium lie at the heart of the hope for obtaining energy from peaceful application of the fusion process.
Since molecules containing deuterium heave chemically like their hydrogen counterparts but can be differentiated from them, it became possible to follow the changes of particular molecules through complicated chemical processes. This was extraordinarily fruitful in studying the mechanisms of chemical reactions and of biochemical processes. George Hevesy, who had already studied the distribution of radioactive lead in plants, immediately understood that the availability of deuterium afforded the first opportunity of studying the fate of a normal biochemical constituent in vivo. Urey well knew Hevesy since his sojourn at Bohr's Institute in Copenhagen, and provided him with a few litres of water containing 0.6 per cent heavy water. With his co-workers Hevesy studied the uptake of water by fish, various other animals, and humans. By monitoring urine levels, they were able to ascertain the average lifetime of a water molecule in the human body and to estimate the total water content, about 1027 molecules.
These achievements further emphasized the importance of Urey’s discovery, for which he was awarded the 1934 Nobel Prize for chemistry.
Geochemistry, Cosmochemistry and Planetary Science
In the 1930s Urey became the leading authority in the new science of isotope chemistry. He was the first editor of the Journal of Chemical Physics published in 1933 by the American Institute of Physics in response to the developing interest in sub-atomic and molecular spectroscopy and structure. Urey remained editor until 1941, establishing the journal as a leader in the newly created field of chemical physics. In 1934 he was appointed to the position of Professor of Chemistry at Columbia University, and from 1939 to 1942 he was the executive officer of the Chemistry Department at Columbia while his scientific work became increasingly concerned with the separation of isotopes.
His discovery of the differences in the chemical and physical property of deuterium compounds led to his broader interest in isotope chemistry and isotope separation. During World War II Urey was appointed to the position of Director of War Research for the Special Alloy Materials (SAM) Laboratories at Columbia, applying his work in uranium isotopes separation and deuterium oxide production to the development of the atomic bomb. He also served as one of three program chiefs in the Manhattan Project. In 1945, at the end of the war, Urey accepted an appointment at the University of Chicago and contributed his efforts to the establishment of the Institute of Nuclear Studies, together with Enrico Fermi, Edward Teller, Leo Szilard, Joseph Mayer, Maria Goeppert Mayer, and others. At Chicago, Urey focused his attention on geochemistry and the problems of the cosmos. In 1947 he published what is probably his most cited paper, ‘”The thermodynamic properties of isotopic substances,” a paper which laid out much of the underpinning of the infant discipline of isotope geochemistry. His work on the measurement of the paleotemperatures of ancient oceans is considered one of the great developments of the earth sciences. This work involved a wide scope of disciplines ranging from Urey's early biological interests to his studies of isotopic fractionation and the history of the earth. For many yeas Urey had been interested in astrophysics and the origins of the solar system. While at Chicago, Urey wrote The Planets: Their Origin and Development, in which he constructed the first systematic and detailed chronology of the origin of the earth, the moon, the meteorites, and the solar system.
In 1958 Urey moved to the University of California's Scripps Institution of Oceanography in La Jolla, where he formed the nucleus of the chemistry program, which later became a leading centre in the field of cosmochemistry. Continuing his investigations of geophysics of the solid earth, geochemistry, meteoritics, the history of the solar system and the origins of life, Urey took an active interest in the United States space program. Associated with the National Aeronautics and Space Administration (NASA), he served as consultant to the Lunar and Planetary Missions Board and was a member of the Planetology Committee. He persuaded NASA to make unmanned missions to the moon and his interest in these matters culminated with the return to earth of the first lunar samples which he personally analysed.
Bibliography
Joseph N. Tatarewicz (2008). Urey, Harold Clayton. Complete Dictionary of Scientific Biography. Vol. 18. Detroit: Charles Scribner's Sons: 943-948
K. P. Cohen, S. K. Runcorn, H. E. Suess and H. G. Thode (1983). Harold Clayton Urey. 29 April 1893-5 January 1981. Biographical Memoirs of Fellows of the Royal Society, Vol. 29: 622-659
James R. Arnold, Jacob Bigeleisen, and Clyde A. Hutchison (1995). Jr. Harold Clayton Urey, Biographical Memoirs of the National Academy of Sciences. http://www.nap.edu/html/biomems/hurey.html
Albert, B. Costa, Urey, Harold Clayton, American National Biography Online. http://www.anb.org/articles/13/13-02119.html?a=1&f=%22Urey%22&d=10&ss=0&q=1
H. G. Thode and Hannes Alfv\'en (1981). Harold C. Urey. Physics Today 34(4): 82-84
Ferdinand G. Brickwedde (1982). Harold Urey and the discovery of deuterium, Physics Today 35(9): 34--39
Charles W. Clark and Joseph Reader (2012). Light, Atoms and Nuclei: The Optical Discovery of Deuterium. Optics and Photonics News 23(5): 36-41
Roger H. Stuewer (1986). The naming of the deuteron. American Journal of Physics 54(3): 206-217