Prof. Dr. Simon van der Meer > Research Profile
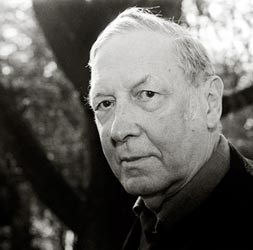
by Roberto Lalli
Simon van der Meer
Nobel Prize in Physics 1984 together with Carlo Rubbia
"for their decisive contributions to the large project, which led to the discovery of the field particles W and Z, communicators of weak interaction".
Simon van der Meer was born in The Hague on 24 November 1925. He grew up in a family strongly committed to education. His father worked as schoolteacher and his mother came from a teacher’s family. Encouraged by his parents’ support, van der Meer attended the gymnasium in his native city. Students of the gymnasium could choose between two alternative programs: the humanities section and the sciences section. Fascinated from an early age by natural sciences, van der Meer opted for the latter and graduated in the sciences section in 1943. He could not enter university immediately after his graduation, though. The German occupation had led to the interruption of any activity in Dutch universities during World War II. Van der Meer managed to pursue his studies by attending the humanity section of the gymnasium till the end of the war in mid-1945. Since his deep interest was already focused on applied physics and technology, especially electronics, Van der Meer became the assistant of his former physics teacher at the gymnasium between 1943 and 1945, his duty being to prepare physics demonstrations.
In the aftermath of World War II, van der Meer enrolled at the prestigious Delft University of Technology, or TU Delft for short. The TU Deft had been founded in 1842 as a Royal Academy and was still the only engineering school in the Netherlands in 1945. Van der Meer selected the newly established course “technical physics,” specializing in measurement and regulation technology under C.J.D.M. Verhagen—a 30-year old engineer, expert in the instrumentation for measuring dynamical quantities.
His training at the TU Deft had a strong influence on his future accomplishments. The curriculum was focused on applied physics, and from these studies van der Meer learnt the importance of practical experience in the resolution of physical problems. On the other hand, the possibility to deepen his knowledge of recent theoretical advancements was highly reduced. Later, van der Meer regretted that he did not have the same intensive physics training as his fellow physicists, but he also recognized that his “slightly amateur approach” helped him in addressing complex issues in a simple and clear fashion.
After having earned his engineering degree in 1952, van der Meer started working at the Philips Natuurkundig Laboratorium (NatLab)— the Dutch branch of the Philips research department. The NatLab was a research department with a strong commitment to basic research following the example of the well-known Bell Telephone Laboratories in the United States. At the NatLab, van der Meer was principally involved in the implementation of high-voltage equipment and electronics for electron microscopes. After four years of experience in this industrial laboratory, van der Meer’s professional life took a new turn when he moved to Geneva to work at the recently founded European Organization for Nuclear Research (CERN)—an internationally coordinated research laboratory devoted to the study of particle physics. He would stay at CERN for the rest of his professional life until his retirement in 1991.
Early Work at CERN
When van der Meer joined the Western European particle physics laboratory in 1956, the CERN staff was involved in the construction of two accelerators: the 600 MeV Synchrocyclotron—which would began operation one year later—and the powerful 28 GeV Proton Synchrotron, which would start up in 1959, becoming the most powerful proton accelerator in the World for a few years.
In his first decade at CERN, Van der Meer’s work mainly concerned technical design for the Proton Synchrotron. Under the leadership of John B. Adams and Colin A. Ramm, van der Meer designed pole-face windings, multipole correction lenses, and their power supplies. Besides this more technical activity, van der Meer was getting interested in the world of elementary particles, as well as in the problematical technological issues concerning their handling. In 1960, he produced his first invention at CERN: the magnetic horn (also known as van der Meer horn). It is a high-current pulsed device that focuses the charged pions into a sharp beam, and, consequently, allows producing a well-focused neutrino beam.
After having dedicated five years of efforts to the construction and implementation of the magnetic horns and the related neutrino flux calculations, in 1965 van der Meer joined for the first time an experimental particle physics group, participating in all the stages of the experiment. A small group headed by the British physicist F. Farley was involved in the design of the second experiment on the measurement of the magnetic moment of the muon, the so-called g-2 muon experiment. The first experiment had ended in 1961, confirming the fractional increase of magnetic moment predicted by Quantum Electrodynamics (QED). The second experiment resulted in a more precise measurement of the magnetic moment, which did not completely agree with the theoretical value calculated in the framework of QED. This unexpected result led to a third experiment, in which van der Meer did not take part. Van der Meer’s work at the second g-2 muon experiment was important for a variety of reasons. From a technical perspective, van der Meer developed insights into the features of accelerators and skills in the construction of storage rings. From the human side, he became acquainted with the various aspects of the lifestyle and mentality of particle physicists.
The Invention of Stochastic Cooling
When van der Meer began working at the g-2 muon experiment, a major step was taken at CERN to increase the energy reached in particle interactions. In 1965, the CERN council formally approved the project of the Intersecting Storage Rings (ISR), which would become the first hadron collider ever built. Proton synchrotrons (PS) accelerate protons into a fixed target. In such a device, most of the kinetic energy acquired by particle beams goes in the forward momentum of the emitted particles in accord with the special relativity laws and the conservation of total linear momentum. A way to overcome such a limitation was to increase the centre-of-mass energy by means of head-on collisions of counter-rotating particles. In this case, the total linear momentum would be relatively low because the particles would have about the same linear momentum in opposite directions, and, consequently, there should be a considerable increase in efficiency. In the ISR project, the PS would feed high-energy protons to two slightly distorted rings intersecting in eight different places, and the centre-of-mass energy of the collisions at these intersections would reach 62 GeV.
In 1967, van der Meer came back to more technical work, becoming responsible for the power supplies of the magnets of the ISR. Van der Meer, however, did not restrict himself to his main task, but provided two important technological advancements for the efficiency of the accelerator under construction. In 1968, he developed a technique for the calibration of the luminosity of colliding beams—the ratio of detected events in a certain time to the interaction cross section. The same year, van der Meer conceived the idea that would gain him the Nobel Prize 16 years later, later called stochastic cooling. According to the Norwegian physicist Kjell Johnsen, who directed the construction of the ISR, van der Meer made this momentous invention under the impression that the ISR would not work as expected. Because of this pessimistic view of the IRS efficiency, van der Meer was seeking a method to increase the luminosity of proton-proton interactions by compressing the protons into finer beams with less energy spread and less angular divergence—a process called cooling in analogy to thermodynamics where disorder is associated with heat. The final result of cooling would be particle bunches compressed in phase space, which could significantly increase the probability of collisions.
In 1966, the Russian physicist Gersch Budker had already conceived a method for cooling protons by employing electron beams to damp the momentum spread of heavy charged particles without any energy loss. Budker’s proposal had the explicit intent to provide a method for increasing the density of protons and antiprotons for making hypothetical proton-antiproton colliders feasible. When the proton-proton collider ISR was under construction in the 1960s, proton-antiproton colliders were believed to be unfeasible because it was impossible to achieve a sufficient density of antiproton beams. Budker’s method remained on the paper for several years and would be tested, successfully, only a decade later.
When van deer Meer conceived a different method, he was only concerned with protons, but he soon recognized that at the ISR proton beams would be dense enough without any cooling. For this reason, van der Meer did not publish his idea immediately. However, some of his colleagues suggested him to develop the method and publish it. The basic idea was to sample the particle motion in a ring and to measure the amount of deviation from the central orbit (the betatron oscillation) with a pick-up and then to correct the deviation with an electromagnetic kicker, which is positioned at a suitable distance from the pick-up and is excited by the amplified pick-up signals. In the ring, there are a large number of particles and, consequently, it is not possible to measure the position of one particle because of the electronic noise. Van der Meer’s technique, however, overcame this difficulty. Instead of considering single particles, one may reason in terms of samples of particles. Then, the pick-up measures the average position of the sample and the kicker corrects this. Calculations led van der Meer to predict that, employing signal amplifiers with a broad bandwidth and low noise, it was possible to compress proton beams with the stochastic cooling in a certain amount of time. However, the same calculation showed that the process would be too slow to be useful in proton accelerators, where the number of particles in the ring is very high (around 10^13). After pressure from the leader of the ISR Instrumentation & Radio
Frequency group, W. Schnell, van der Meer published the proposed methodology as a CERN Internal Report in August 1972 under the title “Stochastic Damping of Betatron Oscillations.”
Schnell and his collaborators studied the feasibility of the stochastic damping (as the method was initially called) at the ISR and, by November 1973, P. Brahma and L. Thorndahl reported the first observation of stochastic cooling of a proton beam at the ISR with a damping time of about four hours.
The Discovery of the W and Z Bosons
In the early 1971, eleven of CERN’s member states approved the construction of the 300-GeV Super Proton Synchrotron (SPS)—the first CERN giant accelerator, whose ring crossed the Swiss-French border. The new accelerator was planned to be a direct competitor to the 500-GeV proton synchrotron that was under construction in Illinois at the recently established National Accelerator Laboratory (after 1974 Fermi National Accelerator Laboratory, or Fermilab for short). From 1971 to 1976, van der Meer was part of the SPS Design Committee as head of the SPS power supplies group.
The CERN staff had not yet had time to begin operation at the SPS, when a new proposal was drafted to radically change the objective of the newly built accelerator. In 1976, C. Rubbia, along with P. McIntyre and D. Cline, proposed a project to convert either the Fermilab Main Ring or the CERN SPS to a proton-antiproton collider in order to detect the intermediate vector bosons predicted by the Glashow-Weinberg-Salam theory of electroweak interactions. In the 1970s, the SU(2)xU(1) gauge theory of electroweak interactions was gaining momentum, and the experimental tests of its predictions were considered among the highest priorities of particle physicists. The charged W and the neutral Z bosons, however, were believed to be very massive (between 50 and 100 GeV), and one needed an amount of centre-of-mass energy much higher than the maximum reached by the two most powerful proton synchrotrons of the period. A conversion of one of these accelerators to a proton-antiproton collider would have allowed reaching energy sufficient to the detection of the heavy bosons.
The project required that the beam of antiprotons was dense enough to allow a sufficient number of collisions to produce heavy bosons. (It was estimated that a heavy boson was produced once every one billion proton-antiproton collisions). While on the other side of the Atlantic it was decided to significantly increase the energy of the Fermilab synchrotron before any further modification of the accelerator, the CERN management resolved to give the project high priority. Before authorizing the conversion of the SPS to a collider, however, it was necessary to confirm that the cooling methods could be really implemented in such a way to produce a dense and compacted beam of antiprotons in a reasonable amount of time.
In 1976, a research group began investigating the technical aspects of the two different methods of electric and stochastic cooling and began constructing a small ring for that purpose. The Initial Cooling Experiment (ICE project), as the test was called, started in December 1977 and in six months provided encouraging results as to the feasibility of the stochastic cooling method, which had several technical advantages with respect to the electric cooling. Van der Meer had an important role in improving the stochastic cooling techniques and in showing that the alternative method was less suitable for antiproton cooling.
In June 1977, the CERN management formally authorized the project for the conversion of the SPS into an antiproton collider. Van der Meer became one of the Project Leaders for the construction of the Antiproton Accumulator (AA)—a small ring for the production of highly compact and dense antiproton beams that had to be eventually injected in the SPS. The AA was ready by mid-1980, only two years after the final approval. Van der Meer personally implemented the computer programs required for the operations of the AA. When the detectors of the two competing experiments designed for the detection of the intermediate vector bosons were ready (the UA1 and UA2 experiments), the density of antiproton beams was still significantly lower than that of proton beams. However, after an accurate study of the background due to proton-gas collisions, the first proton-antiproton collision was detected in July 1981. After more than one year of work on technical improvements, the AA was able to produce antiproton beams intense enough to allow the detection of the charged W particles and, later, of the Z particle. Convincing signatures of all the three particles were first detected by the UA1 collaboration and, slightly later, by the UA2 collaboration. When the public announcement was made that the Z boson had been also detected, the physics community had long been considering the SU(2)xU(1) theory as the correct theory of weak interaction—a recognition that had led to the 1979 Nobel Prize in Physics to the three main contributors to the theory: S. Glashow, S. Weinberg, and A. Salam. No wonder that the CERN findings were immediately considered as a brilliant experimental confirmation of what many took for granted from a theoretical perspective. Only one year later, Van der Meer shared with C. Rubbia the 1984 Nobel Prize "for their decisive contributions to the large project, which led to the discovery of the field particles W and Z, communicators of weak interaction." As the 1984 Nobel Prize press release underlined, the project was the largest for involved number of people ever awarded with a Nobel Prize. The Nobel committee decided to reward two persons of this large cooperative efforts: “Carlo Rubbia, who had and developed the idea, and Simon Van der Meer, whose invention made it feasible.”
From the AA to the AC and Beyond
The impact of the stochastic cooling technique went well beyond its fundamental role in the discovery of the W and Z bosons. The CERN proton-antiproton collider continued to provide several important experimental tests of quantum chromodynamics (QCD), the second pillar of what is called the Standard Model of particle physics. The Fermilab began employing the stochastic cooling technique achieving high luminosity and centre-of-mass energy of 1,8 TeV at the newly built Tetravon in 1986.
The same year, the CERN began the construction of a second ring around the AA to improve the intensity of antiprotons by a factor of ten—a section that was called Antiproton Collector (AC). Van der Meer gave his personal contributions to the design and construction of the AC. His collaborator V. Cohan would later provide a vivid description of the peculiarity of van der Meer’s approach to particle accelerator’s work. “He would work at the highest computational level and then apply himself to the manual tasks with equal dedication.” This mixture of computation and manual ability was also related to a modest personality, which led him to publicly emphasise the contributions given by others.
In his last years of work, van der Meer was considered a precious advisor both at CERN and at Fermilab for the various issues concerning the antiproton accumulation machines and the related software. Although strongly committed to his work, his modesty led him to avoid any further active commitment to CERN activities after the day of his retirement in 1991. Only in rare, very special, occasions he visited the CERN to congratulate on improvements to the high-energy particle physics machines made by his younger colleagues.
Bibliography
Chohan, V. (2012) Simon van der Meer and His Legacy to CERN and Particle Accelerators. arXiv preprint arXiv:1212.4320.
Fitch V. L., & Rosner L. (1995) Elementary Particle Physics in the Second Half of the Twentieth Century. In Brown, L., Pippard, B., & Pais, A. (Eds.). Twentieth Century Physics (Vol. 2). AIP, New York, pp. 635-794.
Galison, P. (1987) How Experiments End. University of Chicago Press, Chicago.
Hoddeson, L. Brown, L. M., Riordan, M. & Dresden, M. (1997) The Rise of the Standard Model: Particle Physics in the 1960s and the 1970s. Cambridge University Press, Cambridge.
Krige, J. (ed.) (1996) History of CERN, vol. 3. Elsevier, Amsterdam.
Press Release: The 1984 Nobel Prize in Physics". Nobelprize.org. Nobel Media AB 2014. Accessed 1 October 2014. <http://www.nobelprize.org/nobel_prizes/physics/laureates/1984/press.html>
Rubbia, C. (1985) Experimental Observation of the Intermediate Vector Bosons W+, W-and Z0. Reviews of Modern Physics 57, pp. 699-722
Sessler A. and Wilson E. (2007) Engines of Discovery: A Century of Particle Accelerators. World Scientific, Singapore.
van der Meer, S. (1985) Simon van der Meer - Biographical. Nobelprize.org. Nobel Media AB 2014. Accessed 1 October 2014. <http://www.nobelprize.org/nobel_prizes/physics/laureates/1984/meer-bio.html>
van der Meer, S. (1985) Stochastic Cooling and the Accumulation of Antiprotons. Reviews of Modern Physics 57, pp. 689-700
Watkins, P. (1986) Story of the W and Z. Cambridge University Press, Cambridge.