Prof. Dr. Max von Laue > Research Profile
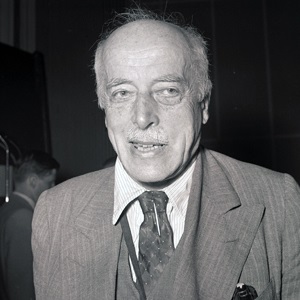
by Luisa Bonolis
Max von Laue
Nobel Prize in Physics 1914
Like Max Planck and Arnold Sommerfeld, Max von Laue defined the period of transition from classical physics to quantum physics. His best-known contribution, the discovery of the diffraction of X-rays by crystals in 1912, was promptly recognised as fundamental for elucidating the nature of both X-rays and crystals. Two years later, when he was awarded the Nobel Prize for Physics, Henry Bragg and his son Lawrence Bragg had already pioneered the new technique of X-ray crystallography, which revolutionised our understanding of the solid state and became an invaluable tool for the ever-expanding field shared by physics, chemistry, crystallography, geology and biology. X-ray spectroscopy, a second spin-off of Laue's discovery, centrally contributed to the development of atomic theory.
An Enthusiastic Supporter of Einstein's Relativity
Max Laue was born on 9 October 1879 in Pfaffendorf and following his father, a civil official of the German military administration, he attended classical schools in different cities. His interest in physics arose already at the age of 12 or 13, when by chance he heard someone talking about the electrolytic deposition of copper from a solution of copper sulphate. He himself wrote in an autobiographical essay: “The impression made upon me by this first encounter with physics was enormous. For several days I went about in deep thought and was good for nothing, so that my mother enquired anxiously what was wrong with me. After having found out, she saw to it that I went to the 'Urania' frequently. This was a society for popularising science in whose premises in the Taubenstrasse in Berlin a great display of physical apparatus was to be found with full explanations and ready to be set in action by pressing a button.” His interest in mathematics and physics continued at the gymnasium, and he read, among others, von Helmholtz's just published Vorträge und Reden.
During the winter 1898-1899, Laue began studying mathematics, physics, and chemistry in Strasbourg, and his enthusiasm for physics continued to increase. In the following year, studying in Göttingen, he was especially influenced by Woldemar Voigt's course and by the reading of Gustav Kirchhoff's published lectures. He thus decided that he wanted to become a theoretical physicist. After two years in Göttingen and a winter semester in Munich, he settled in Berlin in 1902 in order to obtain a degree. Here he encountered Max Planck and began to work on a thesis concerning the application of the principle of conservation of energy in the case of interference phenomena with plane-parallel plates.
He received the degree of Dr. phil. in July 1903 magna cum laude, but he was not yet satisfied with his knowledge, so he added two years of post-doctoral studies in Göttingen. In the autumn of 1905 Planck offered Laue an assistantship at the Institute for Theoretical Physics in Berlin. Laue became Planck's leading and favourite pupil, and the two formed a lifelong friendship. During this period of close contact with Planck, he made outstanding contributions concerning the relation between thermodynamics and radiation, but the most important result of these early years was the beginning of his relationship with Albert Einstein. In September 1905, Einstein reported on his recently published paper on the electrodynamics of moving bodies. After some initial reservations, Laue was captivated by the novel concepts of space and time. In 1907, the first of a series of papers discussing the applications of the special theory of relativity appeared and Laue became one of the first adherents of Einstein's still controversial theory, contributing to its early acceptance. He continued to work on relativity and published the first monograph on this subject, under the title Das Relativitätsprinzip. He expanded it in 1919 with a second volume on the general theory of relativity. These volumes went through several editions, becoming classics in the field.
The Discovery of X-ray Diffraction in Crystals
In 1909 Laue moved to the University of Munich, where Arnold Sommerfeld was then building up a school of theoretical physics. At that time, Sommerfeld was the editor in charge of volume 5 of the monumental Enzyklopädie der Mathematischen Wissenschaften, published in six volumes from 1898 to 1933. His former teacher and friend, the great mathematician Felix Klein in Göttingen, had started this enterprise, which had as its aim a simple and complete review of pure and applied mathematics, including the historical development of mathematical methods from the beginning of the nineteenth century. Volume 5 had been assigned to theoretical physics and Sommerfeld asked Laue to write the chapter on wave optics. Laue's passion for optics -- and especially for the wave theory of light - went back to the lectures he had heard during his student days “from Voigt, Planck and Lummer.” They provided him at that time “with thorough experimental and theoretical knowledge,” so that he was able to cultivate “a special feeling or intuition for wave processes.”
His work for the Encyclopedia required him to express mathematically the action of a diffraction grating on light waves. When incident light is broken up into a large number of individual new sources by the closely spaced, equidistant grooves on a glass plate, the secondary waves from different parts arrive at a point on a screen having travelled different distances. They converge at different points with different phases: where the waves arrive in phase, they reinforce each other and result in a darkened band on a photographic plate, while where they arrive in opposite phase, they will cancel each other. The final result is thus a pattern of light and dark bands on the screen. Laue was extending the mathematics to cover two-dimensional gratings, containing two sets of grooves, when, in February 1912, he discussed with Sommerfeld's doctoral student Paul Ewald problems concerning the passage of long electromagnetic waves through a crystal. Sommerfeld had given Ewald the problem of investigating the effect of an incident electromagnetic wave on an array of isotropically polarisable atoms or molecules arranged on a lattice, in order to understand whether such a system could account for the optical properties of a crystal. Since the end of the previous century, Leonhard Sohncke, Arthur Schoenflies and Evgraf Fedorow had brought the mathematical theory of all possible space-groups of crystals (230) to perfection. The space groups embody all the symmetry properties of the crystal. According to this theory, crystals were supposed to consist of a three-dimensional arrangement of atoms in a lattice, in a regular, repetitive pattern. But no new physical conclusions had evolved from this line of thought, and it played little part in physics and chemistry, remaining unfamiliar to the majority of physicists. But Sohncke had worked in Munich, and many of his models of crystal lattices were still around. Moreover, Paul Groth, a world authority on crystallography, was professor of Mineralogy and Crystallography in Munich, and contributed to keep alive the ideas underlying the theory of space lattices, so that physicists there were acquainted with this area of research and adhered to the hypothesis of the lattice structure of crystals.
Laue, who had learned about these theories, enquired about the likely dimensions of such a lattice, if it existed. During the conversation with Ewald, he was “suddenly struck by the obvious question” of how such electromagnetic waves would behave if their wavelengths were short (much shorter than those of visible light) compared with the spacings between atoms in the crystal lattice. Since his arrival in Munich in 1909, Laue's attention had been constantly drawn - owing to the influence of Röntgen's work at that University and by Sommerfeld's active interest in X-rays and gamma-rays - to the question of their actual nature. It was at that point that his intuition for optics suddenly gave him the answer. He realised that much shorter electromagnetic waves, such as X-rays were supposed to be, must give rise to some kind of interference or diffraction phenomenon in such a medium: “The fact that the lattice constant in crystals is of an order of 10-8 cm was sufficiently known from the analogy with other interatomic distances in solid and liquid substances, and, in addition, this could easily be argued from the density, molecular weight and the mass of the hydrogen atom which, just at that time, had been particularly well determined. The order of X-ray wavelengths was estimated by Wien and Sommerfeld at 10-9 cm. But it would be technically impossible to construct any known form of diffraction grating adequate to demonstrate this diffraction unambiguously.” According to Laue's views, the spacings of the lattice would be the right size to cause the diffraction of X-rays, if indeed such a phenomenon existed. The behaviour of X radiation upon penetrating a crystal should be approximately the same as that of light upon striking a diffraction grating, with which interference phenomena had been studied since Fraunhofer. Laue immediately told Ewald that he anticipated the occurrence of interference phenomena for X-rays transmitted through crystals.
After Röntgen discovered X-rays in 1895, an achievement for which he was the first physicist to be awarded the Nobel Prize in 1901, many efforts had been performed to locate diffraction or interference phenomena in order to solve the question of whether or not they were a form of electromagnetic radiation with a very short wavelength or represented the ejection of small particles. Röntgen himself used a slit to search for X-ray diffraction. Together with electromagnetic radiation, in particular light in the visible spectrum, the mysterious Röntgen rays exhibited polarisation, scattering by matter (already observed by Röntgen himself), and the photoelectric effect. All attempts to display diffraction proved at best inconclusive, though such phenomena were to be expected if X-rays were electromagnetic in nature. From the outset there were supporters of this assumption, and further evidence in support of the wave theory was provided by Charles Barkla, who was later awarded the Nobel Prize in Physics 1917 “for his discovery of the characteristic Röntgen radiation of the elements.” Notwithstanding these major arguments, the wave theory initially did not meet with complete acceptance. For example, experts like Henry Bragg maintained the corpuscular view until 1912, and supporters of that view were able to quote a series of phenomena that, in actual fact, remained inaccessible to explanation by the wave theory.
Laue's proposal of using a crystal as a diffraction grating for X-rays was thus an occasion to test the wave theory. Walter Friedrich, Sommerfeld's recently appointed experimental assistant, immediately expressed his willingness to carry out the relevant test. However, doubts were initially expressed by several physicists about the proposed experiment, considering it hopeless because of the disturbing influence of the temperature motion of the atoms. Moreover, Sommerfeld had involved Friedrich in an experimental check of some theoretical predictions of Sommerfeld's concerning the angular distribution of X-rays coming from an anti-cathode. However, in April 1912 a first experiment, using very simple equipment, was eventually carried out by Friedrich and Paul Knipping, a doctoral candidate, according to Laue's proposal. A copper sulphate crystal irradiated with X-rays yielded regularly ordered dark points on a photographic plate located behind the crystal. The first of what are today called Laue diagrams, betrayed the presence of a considerable number of deflected rays, the lattice spectra that Laue had anticipated. It provided a strong confirmation of the view that X-rays were merely electromagnetic waves, akin to visible light, but of a considerably shorter wavelength. After seeing this result, Laue formulated the mathematical equations for this effect, the three-fold application of the simple condition of diffraction by a linear grating. After this first result, a more elaborate apparatus was constructed in which an accurately oriented and regular crystal could be exposed to a well-collimated X-ray beam. During the following weeks, experimental and theoretical work proved the rightness of the interference theory in connection with lattice periodicities. It was clear that the phenomenon was caused by the crystal structure, since on exposing the pulverised crystal the spots did not appear. All this benefitted not only the wave conception of X-rays by also the lattice theory of crystals. On 4 May 1912 Laue, Friedrich and Knipping announced their successful results in a letter to the Bavarian Academy of Sciences. This first paper included conditions for an X-ray beam of a given wavelength to be diffracted by a periodic array of atoms in a crystal. Einstein himself called Laue's discovery “one of the most beautiful in physics.”
Application of X-ray Interference Phenomena
The considerable interest that had been caused by the initial investigations was soon reflected in a large number of subsequent works. Through the observed Laue diagram, the actual positions of atoms in crystals and the X-ray wavelength could be related, so it was now possible to determine the wavelength of X radiation by using crystals of known structure, as well as to study the structure of the irradiated material.
The experimental research diverged essentially into two branches. On the one hand was the study of crystal lattices, on the other the crystal lattices were used as a tool for spectroscopy in the X-ray range, analysing X-radiation emitted by atoms. This analysis was of great value in the pursuit of an understanding of atomic structure.
The scene shifted to England, where news of the discovery soon reached Henry Bragg, a professor of Physics in Leeds, and his young son Lawrence Bragg, a research student in the Cavendish Laboratory, Cambridge. A study of Laue's photographs suggested to Lawrence Bragg that the radiation was being reflected from sets of atomic parallel planes in the crystal. This was confirmed by reflecting X-rays over a range of angles off a cleavage plane of mica. The condition for a set of planes to scatter in phase an incident beam of wavelength lambda is now known as Braggs Law: lambda=2dsin(theta), where lambda is the wavelength of incident wave, d is the spacing of the planes in the atomic lattice, and theta is the angle between the incident ray and the scattering planes. Other investigators, notably by Georg Wulff in Russia, reached similar conclusions, and Wulff independently formulated Bragg's Law.
It was this concept, rather than the diffraction by a three-dimensional grating put forward by Laue, which Bragg used as the basis for the first X-ray spectrometer, which Bragg constructed, also using his familiarity with characteristic radiation. With the spectrometer, plane after plane of a crystal structure could be investigated and measured out in terms of known wavelengths. Henry Bragg initially used his crystal spectrometer to analyse the radiation from an X-ray source. He found that the entire bremsstrahlung radiation was reflected from a particular crystal face over a range of angles, while superimposed on this was a spectrum of sharp peaks of monochromatic X-rays. Since 1906, Barkla had been discovering the characteristic radiation of different elements. Substances exposed to X rays emit a secondary radiation with a specific penetrative power characteristic of the element concerned. Bragg's observations were greatly extended by Henry Moseley, who commenced a painstakingly accurate determination of the characteristic spectra emitted by various elements, founding the subject of X-ray spectroscopy and introducing the concept of atomic number. After his premature death during World War I, his work was continued, especially in Sweden by Manne Siegbahn and Karl Stenström, on the great majority of chemical elements. This research became of fundamental importance for the further knowledge of the structure of the atom and Manne Siegbahn was awarded the Nobel Prize in Physics 1924 “for his discoveries and research in the field of X-ray spectroscopy”.
Later, the Braggs used the crystal spectrometer to unravel the internal architecture of some crystalline solids, and thus to explain their properties. The first crystal structure the Braggs solved was rock salt. It was surprising to find that there were no discernible individual molecules of sodium chloride, contrary to what all chemists thought at the time. The crystal was one gigantic latticework, each sodium ion surrounded by six equidistant chlorides, each chloride by six equidistant sodiums. Another success was the discovery of the structure of diamond, pure carbon with its atoms linked, as earlier chemists had theorised, to form three-sided pyramids, tetrahedra. Its infinite array of carbon atoms bonded strongly to others in three dimensions explained the origin of its hardness. Diamond and graphite have the same composition, but their structures make them mechanically, chemically and electronically very different, as the structure of graphite later revealed. The Braggs built up a school of scientists who, whether they came from physics or chemistry, were mainly interested in deciphering the atomic arrangement in crystals and to delve deeper than ever before into the mysteries of chemical interaction. Instrumentation and method for measuring X-ray wavelengths with ever-increasing precision and resolution were developed.
The Braggs' achievements further emphasised the extraordinary importance of Laue's discovery, for which he was awarded the 1914 Nobel Prize in Physics. In turn, Laue's work laid the foundation for the methods of X-ray crystallography worked out by Lawrence Bragg during his whole scientific career. The following year the Braggs themselves shared the Nobel Prize for Physics “for their services in the analysis of crystal structure by means of X-rays”. At 25, Lawrence Bragg is still the youngest ever recipient of the Nobel Prize. In 1919, he succeeded Ernest Rutherford as chair of physics at the University of Manchester and in 1938 he again replaced Rutherford, this time as Cavendish professor at the University of Cambridge. Since modern crystallography dawned with X-ray diffraction experiments on crystals, the discipline informed almost every branch of science, also leading to further developments in spectroscopy and solid-state physics and providing a means to understand the structure of complex molecules and materials. After the war, Bragg encouraged his protégés Max Perutz and John Kendrew in their work along the X-ray crystallographic determination of the proteins haemoglobin and myoglobin, a path that would eventually lead them to be jointly awarded the 1962 Nobel Prize for Chemistry. The technique was also instrumental for the elucidation of the molecular structure of penicillin by Dorothy Hodgkin, who was awarded the Nobel Prize in Chemistry 1964 “for her determinations by X-ray techniques of the structures of important biochemical substances”.
The great British tradition of X-ray crystallography initiated by Henry and Lawrence Bragg flourished and after the war financial support allowed the establishment of specific laboratories for the research into the molecular structure of biological systems. The Molecular Biology Unit at Cavendish Laboratory in Cambridge led by Lawrence Braggs attracted researchers who felt that the new field of molecular biology had great promise. Among them were Francis Crick and James Watson who proposed in 1953 a double helix structure for the polynucleotide chain configuration of DNA, an achievement for which they shared the Nobel Prize in Physiology or Medicine with Maurice Wilkins in 1962. They became part of a group formed by nearly 50 scientists who were awarded Nobel Prizes related to X-ray crystallography.
Becoming a Promoter of German Science
In 1912, Laue became Professor of Physics at the University of Zurich and after two years he moved to Frankfurt on Main. During World War I, he was engaged in work on high vacuum tubes used for telephony and wireless communication. In 1917, he accepted the position of deputy director of the new Kaiser Wilhelm Institute for Physics in Berlin, of which Einstein was director and in 1919 he was appointed Professor of Physics at the University of Berlin, where he remained until 1943. Starting from the early 1930s he gave a prominent contribution to the problem of superconductivity, a subject on which he published several papers and a book.
In 1933, when Einstein resigned from the Berlin Academy, the then Vice-President stated that it was no loss to the Academy. As far as is known, Laue was the only member to send in a letter of protest. And when Einstein was dismissed as director of the Kaiser Wilhelm Institute for Physics in Berlin, Laue attacked the new National Socialist government of Adolf Hitler and at the same time defended Einstein' theory of relativity against Nazi apologists such as Phillip von Lenard and Johannes Stark, who labelled it “Jewish science.” In 1945, at the end of World War II Laue was taken prisoner by the Allies and with other German scientists was kept in England to be questioned about Germany's wartime scientific programs. During the last stages of the war, he wrote a book on the history of physics that was later successfully translated into several languages. It was based on his lifelong keen interest in the development of ideas and concepts in physics, to which much of his own reading was devoted. When he returned to Germany in 1946, Laue became acting director of the Max Planck (formerly Kaiser Wilhelm) Institute and professor of physics at the University of Göttingen, as well as consultant to the State Physical-Technical Institute in Berlin. In all these capacities he played a major role in rebuilding German science after the war.
Bibliography
Ewald P. P. (1960) Max von Laue. Biographical Memoirs of Fellows of the Royal Society 6: 134-156
Hermann A. (2008) Laue, Max von. Complete Dictionary of Scientific Biography. Vol. 8. Detroit: Charles Scribner's Sons, pp. 50-53. Gale Virtual Reference Library. http://go.galegroup.com/ps/i.do?id=GALE%7CCX2830902495&v=2.1&u=mpi_vb&it=r&p=GVRL&sw=w&asid=68bb56a920261b5d40f4e942aa1dc1ea
von Laue M. (1915) Concerning the detection of X-ray interferences, Nobel Lecture, November 12. http://www.nobelprize.org/nobel_prizes/physics/laureates/1914/laue-lecture.pdf
Wasson, T. (ed.) (1987) Laue, Max von. In Nobel Prize Winners, H. W. Wilson Company, New York, pp. 599-601