Prof. Dr. Rosalyn Sussman Yalow > Research Profile
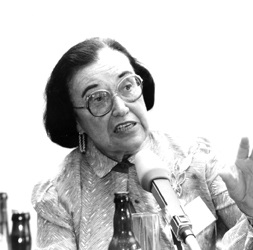
by Luisa Bonolis
Rosalyn Yalow
Nobel Prize in Physiology/Medicine 1977 together with Roger Guillemin and Andrew V. Schally "for the development of radioimmunoassays of peptide hormones".
Rosalyn Sussman Yalow, the second woman after Gerty Radnitz Cori to win the Physiology or Medicine Prize, was also the first American-born woman and the sixth woman to win a Nobel Price in science. She was awarded the Nobel Prize in 1977 for the creation of the radioimmunoassay (RIA), a nuclear technique for quantifying minute amounts of biological substances with in vitro procedures. In using radioactive-labelled material this analytical technique combined the technology of nuclear medicine (tracer technique) and immunology (antigen-antibody binding).
Rosalyn Sussman Yalow earned a Ph.D. in physics with great talent and good fortune in 1945 and had a successful career in medical physics. Considering the barriers to women in science, her achievements are particularly inspiring. She overcame institutional prejudice and demonstrated that women’s accomplishments are limited only by the social and cultural restrictions imposed on them. In this scientific adventure she was joined by Dr. Solomon Berson, until his untimely death in 1972. For twenty years they worked in a spectacular collaboration combining immunology, isotope research, mathematics, and physics. Their invention has had a remarkable impact well beyond earning Sussman Yalow the Nobel Prize. The technique of RIA and its application to a wide variety of biological systems led to important insights in endocrinology, immunology, cardiology, gastroenterology, nephrology, neuroscience, and many other disciplines, thus becoming one of the major accomplishments of medical research in the 20th century, the blueprint for more advanced laboratory techniques.
Becoming a Medical Physicist
Rosalyn Sussman was born in New York City and obtained her early education in the Bronx public schools, developing a lively interest for mathematics and chemistry. She entered Hunter College in 1937, at a time when nuclear physics was developing at a very fast pace, becoming one of the most exciting research fields in physics. She enthusiastically read Eve Curie's just-published biography of her mother Marie, a reading that she ever after recommended to every young aspiring woman scientist: “For me, the most important part of the book was that, in spite of early rejection, she succeeded. It was in common with my background, with my being aggressive.” In 1939 she struggled to have a seat and hear Enrico Fermi giving a colloquium at Columbia University on the recently discovered process for splitting the uranium nucleus. This great novelty was to lead to nuclear weapons as well as to peaceful uses. Also thanks to the development of powerful accelerators that would provide the ready availability of radioisotopes for scientific research and many other applications. Sussman became enthralled with the use of radioactivity and its great peacetime potential for medicine. In January 1941, when Rosalyn Sussman obtained her B. A. with honours, she became the first woman to graduate from Hunter College with a degree in physics. Her parents felt that the most desirable position for her would be as an elementary school teacher. Sussman, however, was courageously dreaming of a career in physics at a time when most people did not really understand the meaning of physics as a research field, even if she was also aware that it was unlikely that good graduate schools would admit (and offer financial support to) a woman interested in science, much less a Jewish woman. In this regard, it is to be recalled that in that same period the physicist and later Nobel laureate Maria Goeppert-Mayer eventually received her first paid teaching appointment, a part-time position at Sarah Lawrence College, after twelve years in the profession.
Sussman was very stubborn and determined, and immediately after graduation, she took work as a secretary to a Columbia University biochemist, and when an unexpected assistantship offer came from the University of Illinois in 1941, she jumped at the chance at pursuing her dream. Encouraged by her professors and also thanks to the beginning of World War II, which was draining American graduate schools of their male students, she was accepted as a teaching assistant and graduate student in the College of Engineering at the University of Illinois, where she was the only woman in a class of 400 students. During the first meeting of the Faculty, the Dean congratulated Rosalyn and informed her she was the first woman there since 1917, during World War I. Rosalyn earned her M. S. in 1942. During her first days at graduate school she had met her future husband, Aaron Yalow, a young student from Syracuse, New York, another aspiring nuclear physicist. Both did their Ph.D. research under the guidance of the renowned nuclear physicist Maurice Goldhaber, later director of Brookhaven National Laboratory. His wife Gertrude was a distinguished physicist, who had no university position because of Illinois' nepotism rules against two relatives working for the same university, a case similar to Maria Goeppert Mayer, who was prevented for a long time to get a position also because of her husband, who was a professor in the same University. The same rule prevented Rosalyn and Aaron Yalow from marrying as long as they were both teaching assistants and technically faculty members. When Aaron got a fellowship elsewhere, they were able to marry in June 1943.
Rosalyn Yalow received her Ph.D in 1945 with an experimental thesis on “Doubly ionized K-shell following radioactive decay.” During her research work for the doctoral dissertation, Yalow spent many hours in the laboratory, where she became skilled at making and using apparatus for the measurement of radioactive substances. After returning to New York City she worked in a research laboratory as assistant engineer - the only woman engineer - and taught physics to veterans enrolled in a pre-engineering course at Hunter College.
It was difficult to find a position in nuclear physics and she was not attracted by high-energy physics with big machines and big teams. By that time, however, she had become intensely interested in medical research and through her husband Aaron she met Dr. Edith Quimby, a leading medical physicist. Yalow volunteered to work in her laboratory to gain research experience in the medical applications of radioisotopes. Quimbly put her in contact with Dr. Gioacchino Failla, the dean of American medical physicists. It was a real turning point in Yalow's career. Radioisotope physics was just coming into use in medicine and Failla found for her a position as consultant to the Radiotherapy Service at the Bronx Veterans Administration (VA) Hospital. There, she established and equipped one of the first radioisotope laboratories in the United States, at a time when the application of radiosotopes as tracer molecules was becoming established. In this technique, one or more of the atoms of the molecule of interest is substituted for an atom of the same chemical element, but of a different radioactive isotope. As the labelled atom has the same number of protons, it will behave in almost exactly the same way as its unlabelled counterpart from the chemical point of view and, with few exceptions, will not interfere with the reaction under investigation. The difference in the number of neutrons, however, means that it will behave differently from a physical point of view. Radioactive isotopes can be detected separately from the other atoms of the same element through their decay products.
Radioisotope research derives from the discovery of artificial radioactivity by Irène and Frédéric Joliot-Curie, the discovery of the neutron by James Chadwick, and the subsequent discovery by Enrico Fermi and his group in Rome that slow neutrons were the most effective way of inducing artificial radioactivity. The development of the cyclotron by Ernest Lawrence (Nobel laureate in 1939) was fundamental for the production of isotopes, even if they were prohibitively expensive for civilian investigators. After the war, nuclear reactors allowed the production of less costly isotopes, both for research and medical treatment. The application of radioisotopes to clinical diagnosis, therapy and investigations opened new ways to investigative medicine. Yalow had been reading a book by the 1943 Nobel Prize laureate George Hevesy, who had pioneered the use of radioactive isotopes as tracers in chemical and physiological processes, greatly advancing the understanding of the chemical nature of life processes. The activity of short-lived radioactive isotopes is detectable as they move through the human body. Hevesy's book thus had an enormous influence on the young Rosayln, helping her to become aware of the potential offered by the application of radioisotopes in medicine.
In 1950, Yalow left her teaching positions at Hunter College and started working full-time at the Bronx VA Hospital, far from the scientific mainstream, as an outsider in every sense. She was working in a new field of physics, and did not have a competence in medicine, so she had to be quite convincing. She developed a radioisotope service for the hospital and started several research projects with its physicians. Her engineering experience proved helpful, because commercial instrumentation was not available at the time and she was able to make or design much of the equipment she needed. Thirty years later she would say, “I'm very proud of the fact that in a sense the VA was the first organization to appreciate the importance of radioisotopes in medicine. The VA provided me with laboratory facilities and the opportunity to grow when I was very young.”
Assaying the Unknown
Medical physics required an interdisciplinary approach, so Yalow began looking for a collaborator to complement her competences. In the spring of 1950, she met Solomon Berson, who was a talented physician and biologist, as well as an accomplished violinist and elegant writer and speaker. Like Yalow, he was an outsider and had faced academic anti-Semitism, but in a more severe form. He was rejected by dozens of medical schools, before he was accepted by the New York University Medical School, even if he had always been an extremely brilliant student. Berson and Yalow complemented each other in many ways. She was a skilled physicist, chemist, and mathematician and had an engineering training. He had a vast clinical knowledge, and supplied the biological insights, physiology, anatomy and clinical medicine. They jointed their competence in a collaborative effort that lasted twenty-two years.
Their early investigations began with an attempt to use radioisotopes to obtain more accurate estimates of blood volume, but their first major contribution dealt with the use of radioiodine in the study of thyroid physiology and the diagnosis of thyroid disease. Because of the ease with which iodine atoms can be attached to tyrosine, one of the 22 amino acids that are used by cells to synthesise proteins, the gamma-radioactive isotopes, Iodine-125 or Iodine-131 can be detected with classical methods of nuclear physics. Injecting radiosotopically labeled substances into the blood and taking samples after the injected material had been mixed uniformly, they developed a method of discerning the quantity of blood cleared of iodine by the thyroid gland per unit of time, providing a quick determination of thyroid activity. They used radiosotopes to assess the distribution of serum proteins in bodily tissues and developed mathematical analyses and experimental methods for studying the rate of removal of serum proteins from the blood stream and determining their rates of synthesis and degradation.
Yalow and Berson were determined to apply their methods to one of the most important classes of small peptides: hormones. They chose insulin as a subject of research because it was the hormone most readily available in a purified form, easy to work with in the laboratory. Insulin is a peptide hormone, produced in the pancreas, and is central to regulating the carbohydrate and fat metabolism in the body, because it causes cells in the skeletal muscles and fat tissue to absorb glucose from the blood.
In 1921, the same year that Rosalyn Yalow was born, Frederick Banting and Charles Best in Toronto, Canada, isolated insulin, preparing an extract from dog pancreas and showed that a compound in the extract was capable of controlling blood glucose concentrations in dogs whose pancreas had been removed. They were later joined by Bertram Collip who was given the task of trying to purify the insulin so that it would be clean enough for testing on humans. The following January, a 14-year old boy was the first person with diabetes to receive insulin. The test was such a success that the news rapidly spread and in 1923 the Nobel Committee decided to award Banting the Nobel Prize in Physiology or Medicine jointly with Rickard Macleod, who had put at disposal his laboratory to perform the experiments supporting the whole project. Feeling that the prize should have been shared between him and Charles Best, Banting decided to share his cash award with him. Macleod, in turn, shared his cash award with Bertram Collip. Two Nobel Prizes were later related to research on insulin. The Nobel Prize in Chemistry was awarded to Frederick Sanger in 1958 “for his work on the structure of proteins, especially that of insulin.” Its three-dimensional structure was determined by Dorothy Hodgkin, who was awarded the Nobel Prize in Chemistry in 1964 “for her determinations by X-ray techniques of the structures of important biochemical substances.”
Yalow was especially interested in insulin, as her husband Aaron was diabetic. Among the endocrine gland disorders, diabetes affects the greatest number of people, making insulin uniquely important. It had been hypothesised that maturity-onset diabetes might not be due to a deficiency of insulin secretion but rather to abnormally rapid degradation of insulin. To test how long insulin remained in the diabetic's system, Berson and Yalow injected patients with tiny amounts of Iodine-131-labelled insulin and took frequent blood samples over the curse of several hours. They measured how fast the hormone was metabolised and disappeared from the plasma of diabetics, using a radiation counter on samples taken at different points in time after the injection. As the supply of insulin from the pancreas is low in diabetic adults and children, general theory held that diabetes was caused by an absolute deficiency of circulating insulin, and thus it had always been assumed that any available insulin would be rapidly utilised by the body. However, Yalow and Berson injected radioactive-labelled insulin to diabetics and non-diabetics and were surprised to find that the tagged insulin took longer to disappear from diabetics than from non-diabetics who had never before been injected insulin.
Why did adult diabetics retain insulin longer than other people? What was the cause of the observed effect? An interesting hint came from the fact that a group of people who had taken insulin as a shock therapy for schizophrenia also retained insulin for an abnormally long time, showing that the cause might be the hormone and not the diabetes.
They suspected that the retarded rate of insulin disappearance was due to binding of labelled insulin to antibodies that the human immune system had developed in response to the administration of exogenous insulin, obtained from the pancreases of pigs and cattle. Insulin bound to a large molecule is harder to excrete or degrade. Antibodies thus made it more difficult for the body to process the hormone and caused it to be cleared from the plasma more slowly.
However, classic immunologic techniques were not adequate for the detection of antibodies, which were likely to be of such low concentration as to be non-precipitating in classical chemical techniques. Radioisotopic methods, however, are of high sensitivity for detection of soluble antigen-antibody complexes.
Yalow and Berson’s results revealed that adult diabetics, unlike diabetic children, do not have an insulin deficiency in their bodies, but are unable to use it to control their blood sugar level. They were actually able to demonstrate the ubiquitous presence of insulin-binding antibodies in insulin-treated subjects, overturning one of the long-held traditions of diabetes: that an insulin molecule was too small to provoke antibody production. This was the first real proof that so small a protein could stimulate an immunologic response. For this reason, manufactured insulin today is genetically engineered to be precisely the same as human insulin. This concept was not easily acceptable to the immunologists of the mid-1950s. Yalow and Berson’s first attempt to publish their observations was rejected by
Science
and initially rejected by the
Journal of Clinical Investigation.
In her Nobel lecture, Yalow presented a copy of the rejection letter, dated September 29, 1955: “They [the experts in the field] believe that you have not demonstrated an antigen-antibody reaction on the basis of adequate criteria, nor that you have definitely proved that a globulin is responsible for insulin binding, nor that insulin is an antigen. The data you present are indeed suggestive but any more positive claim seems unjustifiable at present.” A compromise eventually resulted in acceptance of the paper, but only after the words “insulin antibody” were deleted from the title and, as further recalled by Yalow, only after they documented their conclusion “that the binding globulin was indeed an antibody by showing how it met the definition of antibody given in a standard textbook of bacteriology and immunity.”
Radiommunoassay: a Revolution in Medical Investigation
Their use of radioisotopic techniques for studying the primary reaction of antigen with antibody initiated a revolution in theoretical immunology. But in the course of their investigation on insulin, Berson and Yalow had also come to a starting realisation: the technique they had used to quantify antibodies to a hormone could be used reciprocally to measure concentrations of the antigen, the hormone itself, in this case the circulating insulin. As such it could be seen as the inverse of a radiobinding assay, which quantifies an antibody by use of corresponding antigens. In their 1956 article, eventually accepted by the
Journal of Clinical Investigation
they reported that the binding of labeled insulin to a fixed concentration of antibody is a quantitative function of the amount of insulin present. This observation provided the basis for a method that they called a “radiommunoassay” (RIA), because it used radioactively tagged substances to quantify concentrations of an antigen in solution using antibodies.
The RIA test is simple in principle. Three major substances are present in the reaction: antigen, radiolabelled antigen and correlated specific antibody. The antibody can bind to both labelled and unlabelled antigen. It is kept constant and limited during the test. Radiolabelled antigen possesses the same properties as unlabelled antigen and it is constant, too. The radiolabelled antigen is then mixed with a known amount of its antibody, resulting in formation of a labelled antigen-antibody complex, called bound antigen.
When further unlabelled antigen is added to the mixture, from standards or unknown samples, this causes the new unlabelled antigen to compete with the radiolabelled antigen for a fixed number of antibody or receptor binding sites, leading to more unlabelled antigen-antibody complex and reducing the ratio of antibody-bound radiolabelled antigen to free radiolabelled antigen. The more antigen is present, the less likely is the labelled antigen bound to the antibody, thus the amount of labelled antigen-antibody complex formed is inversely proportional to the antigen originally present in serum. For this reason, this is called a competing depression reaction.
At this point a crucial step is the complete separation between the labelled antigen-antibody complex and the remaining free labelled antigen (separation of bound from unbound labelled antigen). Refined methods had to be developed for handling this phase of the test. The antigen-antibody complexes formed are precipitated using a precipitating reagent (for example a secondary antibody) to
separate bound and free tracer
. Once the antibody-bound antigens are separated from the unbound ones, the radioactivity of both the bound and the remaining free antigen is measured using a gamma counter. With results obtained from a series of standard samples (in which the concentration of the radioactive ligand is always kept constant, but with different concentrations of the inactive ligand), a binding standard (dose-response) curve can be generated. The concentration in the unknown sample may be then read from the standard curve by interpolation.
With RIA, Berson and Yalow provided a means to observe the previously invisible world of antigen-antibody reactions that take place in solution. Now the technique is automated and computerised. At the time, they worked for days preparing solutions in thousands of test tubes, running tests day and night, with no technicians to help, and doing all the work themselves. Radioactive isotopes as markers had been used in medical research before, but Berson and Yalow's work was a specific application of radioactive isotopes in a new investigation area. It was the first time that hormone levels in the blood could be detected by an in vitro assay. As later stated by the Nobel Committee, “[the test] was accomplished by a spectacular combination of immunology, isotope research, mathematics and physics.”
The development of RIA stimulated a revolution in theoretical immunology and actually in all biology. In combining aspects of nuclear medicine and immunology, the method could be used to measure minute substances in blood plasma and other bodily tissues. RIA is actually so incredibly sensitive that it can measure hormones in amounts as small as 1 thousand millionth of a gram. This is like detecting the presence of a teaspoonful of sugar dissolved in a volume of about 90 cubic km of water. Such is the power of radiochemical methods. In addition to its fabulous sensitivity, RIA was a test-tube operation. No radioactivity entered a patient's body. Moreover, RIA worked for virtually every hormone and a variety of other biologically important substances.
The RIA principle, formulated as a spin-off from an unrelated study, which asked a question concerning degradation of insulin in diabetics, transformed into a technique that revolutionised the measurement of protein hormones in the body. It became a new tool helping physicians diagnose conditions that previously escaped detection because established methods were too crude to measure the seemingly small changes in the quantity of hormones that can affect a person's health. RIA had an enormous impact on clinical medicine, leading to major advances in the diagnosis and treatment of the body's major hormone systems, including thyroid function, growth and fertility. In particular, it can be used to make an early diagnosis in babies with underactive thyroid glands, preventing mental retardation. It virtually started a new science - neuroendocrinology, the study of chemical messengers used by the brain to control the body's major hormone systems. Yalow and Berson performed all their work without ever receiving a research grant and even if they recognised that the commercial potential for RIA was enormous, they decided, like the Curies, not to patent their discovery. Instead, they made every effort to get RIA into common use, putting its value to humanity ahead of their own financial interests. The technique was so new and complicated that they spent the three following years developing the concept into a practical test, applying Yalow's physics and chemistry knowledge to the biological problem. In 1960 they presented a methodology for the determination of protein hormones in the blood, the fundamental principle of which utilised the ability of these hormones to stimulate antibody formation. They also began training scientists to use RIA and by the end of the 1960s the field exploded and their technique began to be widely used in laboratories around the world to measure concentrations of hormones and other substances in the body that had previously been too small to detect. RIA can also be employed to detect minute amounts of enzymes, drugs and other substances in bodily fluids or tissues, to screen blood for the hepatitis virus, for the early detection of cancer, and to measure the levels of neurotransmitters, the substances that allow nerve impulses to be transmitted across the gap between nerve cells, or to detect hormones in tissue or plasma. Thanks to RIA transfusion, the hepatitis virus has been eliminated from blood banks.
By using RIA, physicians were able to separate diabetics into two groups: those who lose the ability to make insulin during childhood and those who produce plenty of insulin, but during adulthood lose the ability to use it. The second type of diabetes is today treated with diet - and pills, if necessary. Yalow then used RIA also as a diagnostic tool, using it to measure levels of growth hormone, to track the path of leukemia virus in the stages before actual tumour growth, and to detect classes of new neurotransmitters in the brain. Today, radioimmunoassays are used to measure the concentration of hundreds of hormones, enzymes, vitamins, viruses, and drugs within the human body, even if more advanced methods, notably ELISA, the enzyme-linked immunosorbent assay, a test that uses antibodies and colour change to identify a substance, rely less heavily on radioactive substances and have all but replaced radioimmunoassay in many applications. These new methods are both less dangerous and less costly for scientists.
Between 1968 and 1970 Yalow's work was highly recognised at VA Hospital. She was named acting director of the Radioisotope Service, was appointed director of the Radioimmunoassay Reference Laboratory and director of the Nuclear Medicine Service. After Berson died unexpectedly in 1972, a shocking event for Yalow, she became director of the laboratory, which, at her request, was renamed the Salomon A. Berson Research Laboratory. She also served as research professor in the Department of Medicine at Mt. Sinai, where she was named distinguished service professor in 1974. As her reputation spread, she was elected to the National Academy of Sciences. Next to the Nobel Prize, this is considered the greatest honour that can be given to an American scientist. Between 1974 and 1977 she received five honorary doctorates. In 1976, she became the first woman to be awarded the Albert Lasker Prize for Basic Medical Research. Although extremely prestigious in its own right, the Lasker Prize is generally considered a precursor to the Nobel Prize in Physiology or Medicine.
At 6:45, the morning of October 13, 1977, Yalow was already at work in her study when the telephone rang. She had been awarded half of the Nobel Prize for Physiology or Medicine, “for the development of radioimmunoassays of peptide hormones.” Unfortunately Yalow's late coworker Berson could not share with her the triumph of their long joint activity. The other half was shared jointly by Roger Guillemin and Andrew Schalley, who employed RIA to discover and measure a number of hormones that are released by the brain. In concluding her acceptance speech, Yalow pointed out that “the first telescope opened the heavens; the first microscope opened the world of microbes; radioisotopic methodology, as exemplified by RIA, has shown the potential for opening new vistas in science and medicine.”
Rosalyn Yalow has always worked to further the role of women in science, in particular observing that among the scientists, scholars, and leaders of the world, women are not represented in reasonable proportion to their numbers in the community. She always tried to make women aware of the necessity of working towards the goal of achieving their due status: “... we must believe in us; we must match our aspirations with the guts and determination to succeed; and for those of us who have had the good fortune to move upward, we must feel a personal responsibility to serve as role models and advisors to ease the path for those who come afterwards. We should not ask for reverse discrimination, but simply for equality of opportunity - so that those of us who wish can reach for the stars.”
Bibliography
Glick S. (2011) Rosalyn Sussman Yalow (1921–2011). Nature 474(7353): 580
Kahn R. C. and Ross J. (2012) Rosalyn Sussman Yalow (1921-2011). Proceedings of the National Academy of Sciences of the United States of America 109(3): 669-670
McGrayne S. B. (1993) Rosalyn Sussman Yalow. In Nobel Prize Women in Science. Their Lives, Struggles, and Momentous Discoveries, Carol Publishing Group, pp. 333-355
Opfell O. S. (1986) A sensitive Measure. Rosalyn Yalow. In The Lady Laureates. Women who have won the Nobel Prize, The Scarecrow Press, Inc., Metuchen, N.J., and London, pp. 254-264
Ross J. (2011) A tribute to Rosalyn S. Yalow. The Journal of Clinical Investigation 121(8): 2949-2951
Straus E. (1998) Rosalyn Yalow, Nobel Laureate: Her Life and Work in Medicine: A Biographical Memoir (Plenum, New York)
Sussman Yalow R. (1977) Radioimmunoassay: A probe for the fine structure of biologic systems. Nobel Lecture, December 1977, http://www.nobelprize.org/nobel_prizes/medicine/laureates/1977/yalow-lecture.pdf
Wasson T. (ed.) (1987) Yalow, Rosalyn S. In Nobel Prize Winners, H. W. Wilson Company, New York, pp. 1148-1150
Rosalyn Yalow
Nobel Prize in Physiology/Medicine 1977 together with Roger Guillemin and Andrew V. Schally "for the development of radioimmunoassays of peptide hormones".
Rosalyn Sussman Yalow, the second woman after Gerty Radnitz Cori to win the Physiology or Medicine Prize, was also the first American-born woman and the sixth woman to win a Nobel Price in science. She was awarded the Nobel Prize in 1977 for the creation of the radioimmunoassay (RIA), a nuclear technique for quantifying minute amounts of biological substances with in vitro procedures. In using radioactive-labelled material this analytical technique combined the technology of nuclear medicine (tracer technique) and immunology (antigen-antibody binding).
Rosalyn Sussman Yalow earned a Ph.D. in physics with great talent and good fortune in 1945 and had a successful career in medical physics. Considering the barriers to women in science, her achievements are particularly inspiring. She overcame institutional prejudice and demonstrated that women’s accomplishments are limited only by the social and cultural restrictions imposed on them. In this scientific adventure she was joined by Dr. Solomon Berson, until his untimely death in 1972. For twenty years they worked in a spectacular collaboration combining immunology, isotope research, mathematics, and physics. Their invention has had a remarkable impact well beyond earning Sussman Yalow the Nobel Prize. The technique of RIA and its application to a wide variety of biological systems led to important insights in endocrinology, immunology, cardiology, gastroenterology, nephrology, neuroscience, and many other disciplines, thus becoming one of the major accomplishments of medical research in the 20th century, the blueprint for more advanced laboratory techniques.
Becoming a Medical Physicist
Rosalyn Sussman was born in New York City and obtained her early education in the Bronx public schools, developing a lively interest for mathematics and chemistry. She entered Hunter College in 1937, at a time when nuclear physics was developing at a very fast pace, becoming one of the most exciting research fields in physics. She enthusiastically read Eve Curie's just-published biography of her mother Marie, a reading that she ever after recommended to every young aspiring woman scientist: “For me, the most important part of the book was that, in spite of early rejection, she succeeded. It was in common with my background, with my being aggressive.” In 1939 she struggled to have a seat and hear Enrico Fermi giving a colloquium at Columbia University on the recently discovered process for splitting the uranium nucleus. This great novelty was to lead to nuclear weapons as well as to peaceful uses. Also thanks to the development of powerful accelerators that would provide the ready availability of radioisotopes for scientific research and many other applications. Sussman became enthralled with the use of radioactivity and its great peacetime potential for medicine. In January 1941, when Rosalyn Sussman obtained her B. A. with honours, she became the first woman to graduate from Hunter College with a degree in physics. Her parents felt that the most desirable position for her would be as an elementary school teacher. Sussman, however, was courageously dreaming of a career in physics at a time when most people did not really understand the meaning of physics as a research field, even if she was also aware that it was unlikely that good graduate schools would admit (and offer financial support to) a woman interested in science, much less a Jewish woman. In this regard, it is to be recalled that in that same period the physicist and later Nobel laureate Maria Goeppert-Mayer eventually received her first paid teaching appointment, a part-time position at Sarah Lawrence College, after twelve years in the profession.
Sussman was very stubborn and determined, and immediately after graduation, she took work as a secretary to a Columbia University biochemist, and when an unexpected assistantship offer came from the University of Illinois in 1941, she jumped at the chance at pursuing her dream. Encouraged by her professors and also thanks to the beginning of World War II, which was draining American graduate schools of their male students, she was accepted as a teaching assistant and graduate student in the College of Engineering at the University of Illinois, where she was the only woman in a class of 400 students. During the first meeting of the Faculty, the Dean congratulated Rosalyn and informed her she was the first woman there since 1917, during World War I. Rosalyn earned her M. S. in 1942. During her first days at graduate school she had met her future husband, Aaron Yalow, a young student from Syracuse, New York, another aspiring nuclear physicist. Both did their Ph.D. research under the guidance of the renowned nuclear physicist Maurice Goldhaber, later director of Brookhaven National Laboratory. His wife Gertrude was a distinguished physicist, who had no university position because of Illinois' nepotism rules against two relatives working for the same university, a case similar to Maria Goeppert Mayer, who was prevented for a long time to get a position also because of her husband, who was a professor in the same University. The same rule prevented Rosalyn and Aaron Yalow from marrying as long as they were both teaching assistants and technically faculty members. When Aaron got a fellowship elsewhere, they were able to marry in June 1943.
Rosalyn Yalow received her Ph.D in 1945 with an experimental thesis on “Doubly ionized K-shell following radioactive decay.” During her research work for the doctoral dissertation, Yalow spent many hours in the laboratory, where she became skilled at making and using apparatus for the measurement of radioactive substances. After returning to New York City she worked in a research laboratory as assistant engineer - the only woman engineer - and taught physics to veterans enrolled in a pre-engineering course at Hunter College.
It was difficult to find a position in nuclear physics and she was not attracted by high-energy physics with big machines and big teams. By that time, however, she had become intensely interested in medical research and through her husband Aaron she met Dr. Edith Quimby, a leading medical physicist. Yalow volunteered to work in her laboratory to gain research experience in the medical applications of radioisotopes. Quimbly put her in contact with Dr. Gioacchino Failla, the dean of American medical physicists. It was a real turning point in Yalow's career. Radioisotope physics was just coming into use in medicine and Failla found for her a position as consultant to the Radiotherapy Service at the Bronx Veterans Administration (VA) Hospital. There, she established and equipped one of the first radioisotope laboratories in the United States, at a time when the application of radiosotopes as tracer molecules was becoming established. In this technique, one or more of the atoms of the molecule of interest is substituted for an atom of the same chemical element, but of a different radioactive isotope. As the labelled atom has the same number of protons, it will behave in almost exactly the same way as its unlabelled counterpart from the chemical point of view and, with few exceptions, will not interfere with the reaction under investigation. The difference in the number of neutrons, however, means that it will behave differently from a physical point of view. Radioactive isotopes can be detected separately from the other atoms of the same element through their decay products.
Radioisotope research derives from the discovery of artificial radioactivity by Irène and Frédéric Joliot-Curie, the discovery of the neutron by James Chadwick, and the subsequent discovery by Enrico Fermi and his group in Rome that slow neutrons were the most effective way of inducing artificial radioactivity. The development of the cyclotron by Ernest Lawrence (Nobel laureate in 1939) was fundamental for the production of isotopes, even if they were prohibitively expensive for civilian investigators. After the war, nuclear reactors allowed the production of less costly isotopes, both for research and medical treatment. The application of radioisotopes to clinical diagnosis, therapy and investigations opened new ways to investigative medicine. Yalow had been reading a book by the 1943 Nobel Prize laureate George Hevesy, who had pioneered the use of radioactive isotopes as tracers in chemical and physiological processes, greatly advancing the understanding of the chemical nature of life processes. The activity of short-lived radioactive isotopes is detectable as they move through the human body. Hevesy's book thus had an enormous influence on the young Rosayln, helping her to become aware of the potential offered by the application of radioisotopes in medicine.
In 1950, Yalow left her teaching positions at Hunter College and started working full-time at the Bronx VA Hospital, far from the scientific mainstream, as an outsider in every sense. She was working in a new field of physics, and did not have a competence in medicine, so she had to be quite convincing. She developed a radioisotope service for the hospital and started several research projects with its physicians. Her engineering experience proved helpful, because commercial instrumentation was not available at the time and she was able to make or design much of the equipment she needed. Thirty years later she would say, “I'm very proud of the fact that in a sense the VA was the first organization to appreciate the importance of radioisotopes in medicine. The VA provided me with laboratory facilities and the opportunity to grow when I was very young.”
Assaying the Unknown
Medical physics required an interdisciplinary approach, so Yalow began looking for a collaborator to complement her competences. In the spring of 1950, she met Solomon Berson, who was a talented physician and biologist, as well as an accomplished violinist and elegant writer and speaker. Like Yalow, he was an outsider and had faced academic anti-Semitism, but in a more severe form. He was rejected by dozens of medical schools, before he was accepted by the New York University Medical School, even if he had always been an extremely brilliant student. Berson and Yalow complemented each other in many ways. She was a skilled physicist, chemist, and mathematician and had an engineering training. He had a vast clinical knowledge, and supplied the biological insights, physiology, anatomy and clinical medicine. They jointed their competence in a collaborative effort that lasted twenty-two years.
Their early investigations began with an attempt to use radioisotopes to obtain more accurate estimates of blood volume, but their first major contribution dealt with the use of radioiodine in the study of thyroid physiology and the diagnosis of thyroid disease. Because of the ease with which iodine atoms can be attached to tyrosine, one of the 22 amino acids that are used by cells to synthesise proteins, the gamma-radioactive isotopes, Iodine-125 or Iodine-131 can be detected with classical methods of nuclear physics. Injecting radiosotopically labeled substances into the blood and taking samples after the injected material had been mixed uniformly, they developed a method of discerning the quantity of blood cleared of iodine by the thyroid gland per unit of time, providing a quick determination of thyroid activity. They used radiosotopes to assess the distribution of serum proteins in bodily tissues and developed mathematical analyses and experimental methods for studying the rate of removal of serum proteins from the blood stream and determining their rates of synthesis and degradation.
Yalow and Berson were determined to apply their methods to one of the most important classes of small peptides: hormones. They chose insulin as a subject of research because it was the hormone most readily available in a purified form, easy to work with in the laboratory. Insulin is a peptide hormone, produced in the pancreas, and is central to regulating the carbohydrate and fat metabolism in the body, because it causes cells in the skeletal muscles and fat tissue to absorb glucose from the blood.
In 1921, the same year that Rosalyn Yalow was born, Frederick Banting and Charles Best in Toronto, Canada, isolated insulin, preparing an extract from dog pancreas and showed that a compound in the extract was capable of controlling blood glucose concentrations in dogs whose pancreas had been removed. They were later joined by Bertram Collip who was given the task of trying to purify the insulin so that it would be clean enough for testing on humans. The following January, a 14-year old boy was the first person with diabetes to receive insulin. The test was such a success that the news rapidly spread and in 1923 the Nobel Committee decided to award Banting the Nobel Prize in Physiology or Medicine jointly with Rickard Macleod, who had put at disposal his laboratory to perform the experiments supporting the whole project. Feeling that the prize should have been shared between him and Charles Best, Banting decided to share his cash award with him. Macleod, in turn, shared his cash award with Bertram Collip. Two Nobel Prizes were later related to research on insulin. The Nobel Prize in Chemistry was awarded to Frederick Sanger in 1958 “for his work on the structure of proteins, especially that of insulin.” Its three-dimensional structure was determined by Dorothy Hodgkin, who was awarded the Nobel Prize in Chemistry in 1964 “for her determinations by X-ray techniques of the structures of important biochemical substances.”
Yalow was especially interested in insulin, as her husband Aaron was diabetic. Among the endocrine gland disorders, diabetes affects the greatest number of people, making insulin uniquely important. It had been hypothesised that maturity-onset diabetes might not be due to a deficiency of insulin secretion but rather to abnormally rapid degradation of insulin. To test how long insulin remained in the diabetic's system, Berson and Yalow injected patients with tiny amounts of Iodine-131-labelled insulin and took frequent blood samples over the curse of several hours. They measured how fast the hormone was metabolised and disappeared from the plasma of diabetics, using a radiation counter on samples taken at different points in time after the injection. As the supply of insulin from the pancreas is low in diabetic adults and children, general theory held that diabetes was caused by an absolute deficiency of circulating insulin, and thus it had always been assumed that any available insulin would be rapidly utilised by the body. However, Yalow and Berson injected radioactive-labelled insulin to diabetics and non-diabetics and were surprised to find that the tagged insulin took longer to disappear from diabetics than from non-diabetics who had never before been injected insulin.
Why did adult diabetics retain insulin longer than other people? What was the cause of the observed effect? An interesting hint came from the fact that a group of people who had taken insulin as a shock therapy for schizophrenia also retained insulin for an abnormally long time, showing that the cause might be the hormone and not the diabetes.
They suspected that the retarded rate of insulin disappearance was due to binding of labelled insulin to antibodies that the human immune system had developed in response to the administration of exogenous insulin, obtained from the pancreases of pigs and cattle. Insulin bound to a large molecule is harder to excrete or degrade. Antibodies thus made it more difficult for the body to process the hormone and caused it to be cleared from the plasma more slowly.
However, classic immunologic techniques were not adequate for the detection of antibodies, which were likely to be of such low concentration as to be non-precipitating in classical chemical techniques. Radioisotopic methods, however, are of high sensitivity for detection of soluble antigen-antibody complexes.
Yalow and Berson’s results revealed that adult diabetics, unlike diabetic children, do not have an insulin deficiency in their bodies, but are unable to use it to control their blood sugar level. They were actually able to demonstrate the ubiquitous presence of insulin-binding antibodies in insulin-treated subjects, overturning one of the long-held traditions of diabetes: that an insulin molecule was too small to provoke antibody production. This was the first real proof that so small a protein could stimulate an immunologic response. For this reason, manufactured insulin today is genetically engineered to be precisely the same as human insulin. This concept was not easily acceptable to the immunologists of the mid-1950s. Yalow and Berson’s first attempt to publish their observations was rejected by
Science
and initially rejected by the
Journal of Clinical Investigation.
In her Nobel lecture, Yalow presented a copy of the rejection letter, dated September 29, 1955: “They [the experts in the field] believe that you have not demonstrated an antigen-antibody reaction on the basis of adequate criteria, nor that you have definitely proved that a globulin is responsible for insulin binding, nor that insulin is an antigen. The data you present are indeed suggestive but any more positive claim seems unjustifiable at present.” A compromise eventually resulted in acceptance of the paper, but only after the words “insulin antibody” were deleted from the title and, as further recalled by Yalow, only after they documented their conclusion “that the binding globulin was indeed an antibody by showing how it met the definition of antibody given in a standard textbook of bacteriology and immunity.”
Radiommunoassay: a Revolution in Medical Investigation
Their use of radioisotopic techniques for studying the primary reaction of antigen with antibody initiated a revolution in theoretical immunology. But in the course of their investigation on insulin, Berson and Yalow had also come to a starting realisation: the technique they had used to quantify antibodies to a hormone could be used reciprocally to measure concentrations of the antigen, the hormone itself, in this case the circulating insulin. As such it could be seen as the inverse of a radiobinding assay, which quantifies an antibody by use of corresponding antigens. In their 1956 article, eventually accepted by the
Journal of Clinical Investigation
they reported that the binding of labeled insulin to a fixed concentration of antibody is a quantitative function of the amount of insulin present. This observation provided the basis for a method that they called a “radiommunoassay” (RIA), because it used radioactively tagged substances to quantify concentrations of an antigen in solution using antibodies.
The RIA test is simple in principle. Three major substances are present in the reaction: antigen, radiolabelled antigen and correlated specific antibody. The antibody can bind to both labelled and unlabelled antigen. It is kept constant and limited during the test. Radiolabelled antigen possesses the same properties as unlabelled antigen and it is constant, too. The radiolabelled antigen is then mixed with a known amount of its antibody, resulting in formation of a labelled antigen-antibody complex, called bound antigen.
When further unlabelled antigen is added to the mixture, from standards or unknown samples, this causes the new unlabelled antigen to compete with the radiolabelled antigen for a fixed number of antibody or receptor binding sites, leading to more unlabelled antigen-antibody complex and reducing the ratio of antibody-bound radiolabelled antigen to free radiolabelled antigen. The more antigen is present, the less likely is the labelled antigen bound to the antibody, thus the amount of labelled antigen-antibody complex formed is inversely proportional to the antigen originally present in serum. For this reason, this is called a competing depression reaction.
At this point a crucial step is the complete separation between the labelled antigen-antibody complex and the remaining free labelled antigen (separation of bound from unbound labelled antigen). Refined methods had to be developed for handling this phase of the test. The antigen-antibody complexes formed are precipitated using a precipitating reagent (for example a secondary antibody) to
separate bound and free tracer
. Once the antibody-bound antigens are separated from the unbound ones, the radioactivity of both the bound and the remaining free antigen is measured using a gamma counter. With results obtained from a series of standard samples (in which the concentration of the radioactive ligand is always kept constant, but with different concentrations of the inactive ligand), a binding standard (dose-response) curve can be generated. The concentration in the unknown sample may be then read from the standard curve by interpolation.
With RIA, Berson and Yalow provided a means to observe the previously invisible world of antigen-antibody reactions that take place in solution. Now the technique is automated and computerised. At the time, they worked for days preparing solutions in thousands of test tubes, running tests day and night, with no technicians to help, and doing all the work themselves. Radioactive isotopes as markers had been used in medical research before, but Berson and Yalow's work was a specific application of radioactive isotopes in a new investigation area. It was the first time that hormone levels in the blood could be detected by an in vitro assay. As later stated by the Nobel Committee, “[the test] was accomplished by a spectacular combination of immunology, isotope research, mathematics and physics.”
The development of RIA stimulated a revolution in theoretical immunology and actually in all biology. In combining aspects of nuclear medicine and immunology, the method could be used to measure minute substances in blood plasma and other bodily tissues. RIA is actually so incredibly sensitive that it can measure hormones in amounts as small as 1 thousand millionth of a gram. This is like detecting the presence of a teaspoonful of sugar dissolved in a volume of about 90 cubic km of water. Such is the power of radiochemical methods. In addition to its fabulous sensitivity, RIA was a test-tube operation. No radioactivity entered a patient's body. Moreover, RIA worked for virtually every hormone and a variety of other biologically important substances.
The RIA principle, formulated as a spin-off from an unrelated study, which asked a question concerning degradation of insulin in diabetics, transformed into a technique that revolutionised the measurement of protein hormones in the body. It became a new tool helping physicians diagnose conditions that previously escaped detection because established methods were too crude to measure the seemingly small changes in the quantity of hormones that can affect a person's health. RIA had an enormous impact on clinical medicine, leading to major advances in the diagnosis and treatment of the body's major hormone systems, including thyroid function, growth and fertility. In particular, it can be used to make an early diagnosis in babies with underactive thyroid glands, preventing mental retardation. It virtually started a new science - neuroendocrinology, the study of chemical messengers used by the brain to control the body's major hormone systems. Yalow and Berson performed all their work without ever receiving a research grant and even if they recognised that the commercial potential for RIA was enormous, they decided, like the Curies, not to patent their discovery. Instead, they made every effort to get RIA into common use, putting its value to humanity ahead of their own financial interests. The technique was so new and complicated that they spent the three following years developing the concept into a practical test, applying Yalow's physics and chemistry knowledge to the biological problem. In 1960 they presented a methodology for the determination of protein hormones in the blood, the fundamental principle of which utilised the ability of these hormones to stimulate antibody formation. They also began training scientists to use RIA and by the end of the 1960s the field exploded and their technique began to be widely used in laboratories around the world to measure concentrations of hormones and other substances in the body that had previously been too small to detect. RIA can also be employed to detect minute amounts of enzymes, drugs and other substances in bodily fluids or tissues, to screen blood for the hepatitis virus, for the early detection of cancer, and to measure the levels of neurotransmitters, the substances that allow nerve impulses to be transmitted across the gap between nerve cells, or to detect hormones in tissue or plasma. Thanks to RIA transfusion, the hepatitis virus has been eliminated from blood banks.
By using RIA, physicians were able to separate diabetics into two groups: those who lose the ability to make insulin during childhood and those who produce plenty of insulin, but during adulthood lose the ability to use it. The second type of diabetes is today treated with diet - and pills, if necessary. Yalow then used RIA also as a diagnostic tool, using it to measure levels of growth hormone, to track the path of leukemia virus in the stages before actual tumour growth, and to detect classes of new neurotransmitters in the brain. Today, radioimmunoassays are used to measure the concentration of hundreds of hormones, enzymes, vitamins, viruses, and drugs within the human body, even if more advanced methods, notably ELISA, the enzyme-linked immunosorbent assay, a test that uses antibodies and colour change to identify a substance, rely less heavily on radioactive substances and have all but replaced radioimmunoassay in many applications. These new methods are both less dangerous and less costly for scientists.
Between 1968 and 1970 Yalow's work was highly recognised at VA Hospital. She was named acting director of the Radioisotope Service, was appointed director of the Radioimmunoassay Reference Laboratory and director of the Nuclear Medicine Service. After Berson died unexpectedly in 1972, a shocking event for Yalow, she became director of the laboratory, which, at her request, was renamed the Salomon A. Berson Research Laboratory. She also served as research professor in the Department of Medicine at Mt. Sinai, where she was named distinguished service professor in 1974. As her reputation spread, she was elected to the National Academy of Sciences. Next to the Nobel Prize, this is considered the greatest honour that can be given to an American scientist. Between 1974 and 1977 she received five honorary doctorates. In 1976, she became the first woman to be awarded the Albert Lasker Prize for Basic Medical Research. Although extremely prestigious in its own right, the Lasker Prize is generally considered a precursor to the Nobel Prize in Physiology or Medicine.
At 6:45, the morning of October 13, 1977, Yalow was already at work in her study when the telephone rang. She had been awarded half of the Nobel Prize for Physiology or Medicine, “for the development of radioimmunoassays of peptide hormones.” Unfortunately Yalow's late coworker Berson could not share with her the triumph of their long joint activity. The other half was shared jointly by Roger Guillemin and Andrew Schalley, who employed RIA to discover and measure a number of hormones that are released by the brain. In concluding her acceptance speech, Yalow pointed out that “the first telescope opened the heavens; the first microscope opened the world of microbes; radioisotopic methodology, as exemplified by RIA, has shown the potential for opening new vistas in science and medicine.”
Rosalyn Yalow has always worked to further the role of women in science, in particular observing that among the scientists, scholars, and leaders of the world, women are not represented in reasonable proportion to their numbers in the community. She always tried to make women aware of the necessity of working towards the goal of achieving their due status: “... we must believe in us; we must match our aspirations with the guts and determination to succeed; and for those of us who have had the good fortune to move upward, we must feel a personal responsibility to serve as role models and advisors to ease the path for those who come afterwards. We should not ask for reverse discrimination, but simply for equality of opportunity - so that those of us who wish can reach for the stars.”
Bibliography
Glick S. (2011) Rosalyn Sussman Yalow (1921–2011). Nature 474(7353): 580
Kahn R. C. and Ross J. (2012) Rosalyn Sussman Yalow (1921-2011). Proceedings of the National Academy of Sciences of the United States of America 109(3): 669-670
McGrayne S. B. (1993) Rosalyn Sussman Yalow. In Nobel Prize Women in Science. Their Lives, Struggles, and Momentous Discoveries, Carol Publishing Group, pp. 333-355
Opfell O. S. (1986) A sensitive Measure. Rosalyn Yalow. In The Lady Laureates. Women who have won the Nobel Prize, The Scarecrow Press, Inc., Metuchen, N.J., and London, pp. 254-264
Ross J. (2011) A tribute to Rosalyn S. Yalow. The Journal of Clinical Investigation 121(8): 2949-2951
Straus E. (1998) Rosalyn Yalow, Nobel Laureate: Her Life and Work in Medicine: A Biographical Memoir (Plenum, New York)
Sussman Yalow R. (1977) Radioimmunoassay: A probe for the fine structure of biologic systems. Nobel Lecture, December 1977, http://www.nobelprize.org/nobel_prizes/medicine/laureates/1977/yalow-lecture.pdf
Wasson T. (ed.) (1987) Yalow, Rosalyn S. In Nobel Prize Winners, H. W. Wilson Company, New York, pp. 1148-1150