Click Chemistry and Medicine
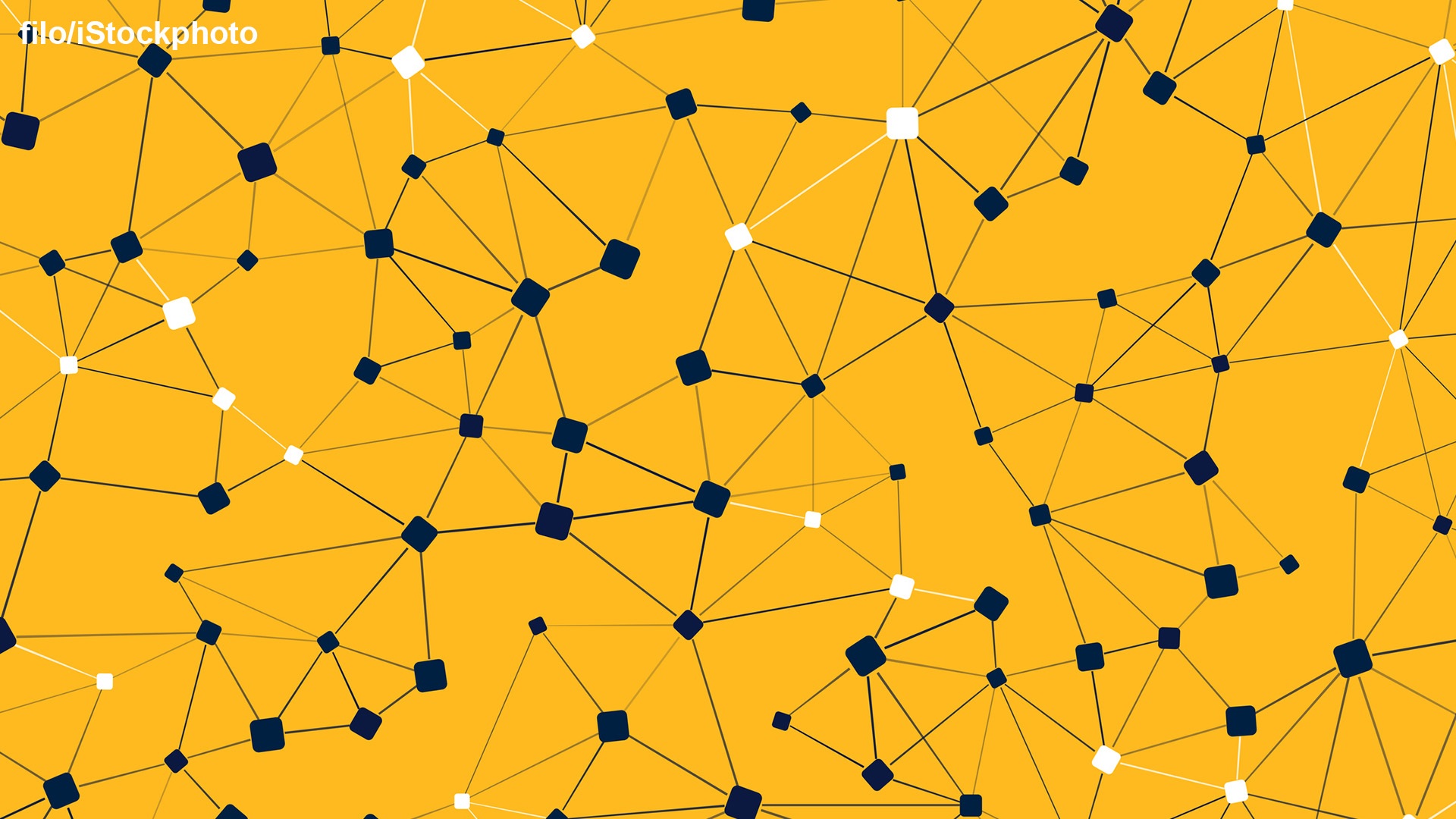
By Hanna Kurlanda-Witek
In 1909, the future Nobel Laureate Theodore William Richards wrote, “Chemistry (...) holds the key which alone can unlock the gate to really fundamental knowledge of the hidden causes of health and disease.” Well over a century later, there is so much that we know about chemistry, and yet still so many problems waiting to be solved. “(...) The human body is a wonderfully intricate chemical machine; and its health and illness, its life and death, are essentially connected with the coordination of a variety of complex chemical changes,” wrote Richards. The chemical complexity of the human body is one matter, and synthesising chemical compounds that can eradicate disease without harming the body, yet another.
In this Lindau lecture fragment, Nobel Laureate Ryoji Noyori explains the importance of chemistry in our lives:
(00:05:34 - 00:06:41)
Chemical reactions taking place under the fume hood are the first step towards finding efficient drugs. During his last Lindau Nobel Laureate Meeting in 2021, the late Robert H. Grubbs described how olefin metathesis catalysed by ruthenium eventually led to the development of a drug for hepatitis C:
(00:20:00 - 00:30:00)
The Search for New Reactions
Chemical reactions have often been discovered by accident, with chemists then determining what their uses could be, particularly in real-world applications. Here, Sir Derek H. R. Barton describes several chemical reactions, which took chemists by surprise. At the end of the fragment, he mentions K. Barry Sharpless, the future recipient of two Nobel Prizes in Chemistry, including one for developing click chemistry.
(00:00:24 - 00:05:07)
Nowadays, chemists have a more focused and intentional approach to finding new reactions and molecules. As Sharpless said in his 2022 Nobel Lecture, the magic of chemistry lies in not making compounds, but properties. Roger Tsien raised the issue in his lecture, not only emphasising the applications of chemistry in biological systems and understanding of the science at a deeper level, but also the need for creativity:
(00:01:20 - 00:03:07)
Click Chemistry: Bringing Simplicity to the Lab
During his lecture at Lindau in 1989, Nobel Laureate Herbert C. Brown encouraged fellow chemists to get out of the easy chair and go to the lab; “Invariably something comes up that opens up a whole new door to a new field of chemistry.”
Such was the case with click chemistry, a term which was introduced by K. Barry Sharpless and colleagues in a paper in 2001. They postulated that molecules found in nature were built with carbon-heteroatom bonds, and this should be the preferred method of synthesising organic molecules, rather than focusing on carbon-carbon (C-C) bonds, which are very difficult to make in the lab and form many undesirable by-products.
Sharpless suggested the formation of molecular frameworks using heteroatom links (C-X-C), where the X could be nitrogen, oxygen, sulphur, or other atoms. These reactions are much easier to synthesise for the chemist, and only a handful of reliable reactions are enough to link together many different molecules, leading to countless new products. Sharpless called these types of reactions “click reactions”, as the molecules simply snapped into place, not unlike Lego blocks.
Soon after, Sharpless and Danish chemist Morten Meldal independently published a description of the first click reaction, the synthesis of a triazole (a compound with a ring of three nitrogen atoms and two carbon atoms) from an azide and an alkyne, using copper to speed up the reaction. This became known as the Cu-Catalyzed Azide-Alkyne Cycloaddition (or CuAAC), a long name for a reaction known universally today for binding together different molecules.
(00:09:15 - 00:11:10)
What makes click reactions stand out is not just their simplicity, robustness and speed, but also their “atom economy”; the atoms of the reactants end up in the product. Moreover, click reactions aren’t confined to a flask in a lab−they can take place in different mediums; in water, on the surface of compounds, and, as Carolyn Bertozzi demonstrated in 2004, in living cells.
Chemistry Probing the Unexplored Areas of Cell Biology
In the introduction of her Nobel Lecture, Carolyn R. Bertozzi noted that unmet needs in biology inspire developments in chemistry. In the 1990s, when scientists put recently developed techniques in molecular biology to use in order to gain a better understanding of proteins and DNA, Bertozzi chose to focus on cell carbohydrates.
Every cell is coated with a mixture of carbohydates, which are also linked to proteins and lipids, and this external cell layer is known as a glycocalyx. For many years, the glycocalyx was thought to be simply a way of protecting the cell, but scientists began to suppose that it had many more functions. Bertozzi wanted to image complex carbohydrates, known as glycans, by attaching a fluorescent probe to them. Importantly, the probe had to be neutral for the cell and couldn’t react with molecules other than glycans.
Bertozzi succeeded in finding the right chemical probe to image the glycan by delving into the history books. In 1907, Hermann Staudinger, a German organic chemist, discovered that an azide can react with a phosphine, forming an aza-ylide. Bertozzi and her students modified the so-called Staudinger reaction, and demonstrated that azides can be used in living cells without disturbing any of the biochemical processes taking place within the cell − a property that became the cornerstone of bioorthogonal chemistry. Bertozzi’s lab even performed the reaction in living mice, but the drawback of these experiments was that they were very slow. The Staudinger ligation, as the modified version of the original reaction is known, took hours, sometimes up to a day to work.
Click Chemistry in Living Organisms
After the publication of the first click reaction, Bertozzi realised this might help speed up the Staudinger ligation in the cell. But there was an obstacle; azide-alkyne cycloaddition uses copper as a catalyst, and copper is toxic to living organisms.
This toxicity was explained by Morten Meldal during his lecture in Lindau in 2023:
(00:12:25 - 00:13:32)
The group had to find a way to perform this reaction without metal catalysts, and at room temperature.
The answer was found in another historical reaction, discovered by Georg Wittig in 1954. In the early 1960s, Wittig reported that azide-alkyne cycloaddition was possible when the alkyne was modified into a ring shape. The strain of the ring would cause the cycloaddition to take place very quickly. By attaching a fluorescent green molecule to the ring-shaped alkyne, and introducing a molecule consisting of a sugar and an azide directly into the cell, Bertozzi showed that a click reaction between the azide and the alkyne could take place in glycans. The fluorescent green molecule was attached to the ensuing triazole, making it possible to visualise the glycocalyx using fluorescence microscopy. This was the first “copper-free” click reaction − the strain-promoted alkyne-azide cycloaddition (SPAAC), described ini this lecture fragment by Morten Meldal:
(00:20:43 - 00:21:32)
Click Reactions in Medicine: Only the Beginning
The last two decades have seen an incredible amount of research in click chemistry, with hundreds of publications and new uses reported every year. There are many alternative bioorthogonal reactions that have been developed since SPAAC was first described.
As Bertozzi explained during her Nobel Lecture, click chemistry in biological systems was developed as a tool for basic research, yet now the aim is “to create new medicines and diagnostic strategies for human disease.”
One of the most fruitful areas of click chemistry used in drug development is the synthesis of antibody-drug conjugates. These are cancer drugs that combine monoclonal antibodies targeted at particular tumour cells with a specific cell-killing agent; the two conjugates are linked together with the use of click chemistry. As of 2022, 14 antibody-drug conjugates have been approved for use by the US Food and Drug Administration (FDA) and hundreds of others are in clinical trials.
In general, click chemistry in therapeutics is used to facilitate drug delivery in the cell, for example as click-activated protodrugs, which become active once they are in the cell. Click reactions can also be used in biomolecular tracking or signalling, a critical step in the early phase of drug design, as the exact location of the active agent has to be visualised in the cell. Other applications are the delivery of radionuclides, and the modification of nucleic acids and proteins. Click reactions incorporated into drug design will generate new classes of therapeutics for a wide range of diseases, such as cardiovascular and autoimmune diseases.
During his lecture, Morten Meldal mentioned other click reactions in medicine, namely drugs used in fibrosis and molecular robots:
(00:11:11 - 00:12:25)
Precision Medicine
Drug development is a laborious process, and demonstrating the safety and efficacy of new medicines through clinical trials is the foundation of bringing them to the public. But for most of the history of medicine, including the 20th century when many life-saving chemicals were synthesised in the lab, drug development was based on a trial-and-error approach.
(00:06:41 - 00:09:03)
The 20th century has witnessed a number of milestones in the development of therapeutics, such as the discovery of penicillin and other antibiotics, and the synthesis of vaccines. As Nobel Laureate Aaron Ciechanover explained here, by the 1970s the preferred method of developing drugs was to screen large libraries of compounds to find the one that would match the illness:
(00:12:23 - 00:14:58)
Click chemistry is aligned with the fast-expanding field of precision medicine, which enables specific therapies for the patient, replacing one-size-fits-all therapeutic models:
(00:11:13 - 00:15:30)
Nobel Laureate Ben L. Feringa expressed the need to design new molecules for medicine and the necessity to “dock molecules in the right spot”− this is exactly the way click reactions work in the human body:
(00:25:18 - 00:29:14)
While the synthesis of new chemical compounds is vital for precision medicine, the affordability of these therapies is an unresolved problem. The model of drug development described by Nobel Laureate Ryoji Noyori in 2009 is unfortunately still the norm today. Developing new drugs is an expensive and time-consuming process, often resulting in clinical trials with a high drop-out rate:
(00:17:44 - 00:18:56)
Will precison medicine reverse this system? In a recent lecture, Aaron Ciechanover said he thinks targeted therapies will continue to be more expensive, as there will be a lower number of patients who will be the recipients of the drug:
(00:38:11 - 00:41:27)
It’s more and more likely that eventually governments around the world will have to play an important role in making novel treatments accessible to the general population, with the aim of making precision medicine a routine part of the healthcare system. In the meantime, a powerful tool is set to change how chemical compounds are screened for use as potential medicines.
AI Facilitating Complex Data Analysis in Biochemistry
The last several years have seen a progressive development of Artificial Intelligence (AI), including deep learning and machine learning algorithms, in many areas of scientific research. This is particularly evident in fields that use a large amount of data, such as genomics and proteomics, where analysis of complex data would be almost impossible to compute manually. The fast processing times and greater efficiency of AI algorithms have opened up new avenues of research and the possibility to design experiments differently.
AI is already proving itself useful in selecting candidates for new click chemistry reactions, and this can be a valid pathway for designing molecules and predicting their efficiency in drug development.
During this panel discussion on AI in chemistry, Nobel Laureate Arieh Warshel answered a Young Scientist's question about how AI can help with enzyme design:
(00:44:16 - 00:47:18)
It may well be that in a few years time, scientists will be wondering how they were able to do research without AI, but at this stage, the results still need to be verified and tested by humans. As Warshel noted during the panel discussion, “AI gives you hints,” by screening data and pinpointing which reactions can work, but their practicality has to be validated experimentally. In the panel discussion on personalised medicine, Aaron Ciechanover also underlined that it’s still necessary “to go back to the bench”:
(00:56:11 - 00:58:12)
Not Just Chemists On Their Own
The field of click chemistry is just over 20 years old, yet with all its predicted applications, not only in medical science, but also in materials science and polymer chemistry, it is still in its infancy. As with most areas of scientific research, collaboration between scientists, for example organic chemists, biomolecular engineers and bioinformaticians, will be the driving factor in how quickly practical applications of click chemistry will reach the market. During a panel discussion on catalysis and green chemistry, Nobel Laureate Sir David W.C. MacMillan explained why it’s essential to blur the lines between the defined categories of science:
(00:28:44 - 00:32:25)
In 1986, Sir Derek H. R. Barton concluded his lecture at Lindau by saying that there’s much to be done in chemistry, and “anyone can make a new reaction.” Fortunately, this is still true.
(00:34:57 - 00:35:54)
References:
Badria A. Click Chemistry: A Promising Tool for Building Hierarchical Structures. Polymers 2022, 14(9), 4077; https://doi.org/10.3390/polym14194077.
Fantoni NZ, El-Sagheer AH, Brown T. A Hitchhiker’s Guide to Click-Chemistry with Nucleic Acids. Chem Rev 2021, 121(12):7122-7154. https://doi.org/10.1021/acs.chemrev.0c00928
Johnson KB, Wei WQ, Weeraratne D, et al. Precision Medicine, AI, and the Future of Personalized Health Care. Clin Transl Sci 2021 Jan; 14(1):86-93. doi: 10.1111/cts.12884
Kolb HC, Finn MG, Sharpless KB. Click Chemistry: Diverse Chemical Function From a Few Good Reactions. Angew Chem Int Ed Engl. 2001 Jun 1;30(11):2004-2021; doi: 10.1002/1521-3773(20010601)40:11<2004::AID-ANIE2004>3.0.CO;2-5.
Kondengadan SM, Bansal S, Yang C, et al. Click Chemistry and Drug Delivery: A Bird’s-Eye View. Acta Pharm Sin B. 2023 May; 13(5):1990-2016; doi: 10.1016/j.apsb.2022.10.015.
Stuyver T, Coley CW. Machine Learning-Guided Computational Screening of New Candidate Reactions with High Bioorthogonal Click Potential. Chemistry−A European Journal 2023 May 29(28); https://doi.org/10.1002/chem.202300387.
Tong JTW, Harris PWR, Brimble MA, Kavianinia I. An Insight into FDA Approved Antibody-Drug Conjugates for Cancer Therapy. Molecules 2021 Oct; 26(19):5847.
Further Reading:
https://www.nobelprize.org/uploads/2022/10/popular-chemistryprize2022-2.pdf
https://www.nobelprize.org/prizes/chemistry/2022/summary/
https://www.mcgill.ca/oss/article/medical-did-you-know/lesson-nature-what-click-chemistry-and-why-it-won-nobel-prize
https://www.theatlantic.com/magazine/archive/1909/01/modern-chemistry-and-medicine/529875/