Life of Proteins
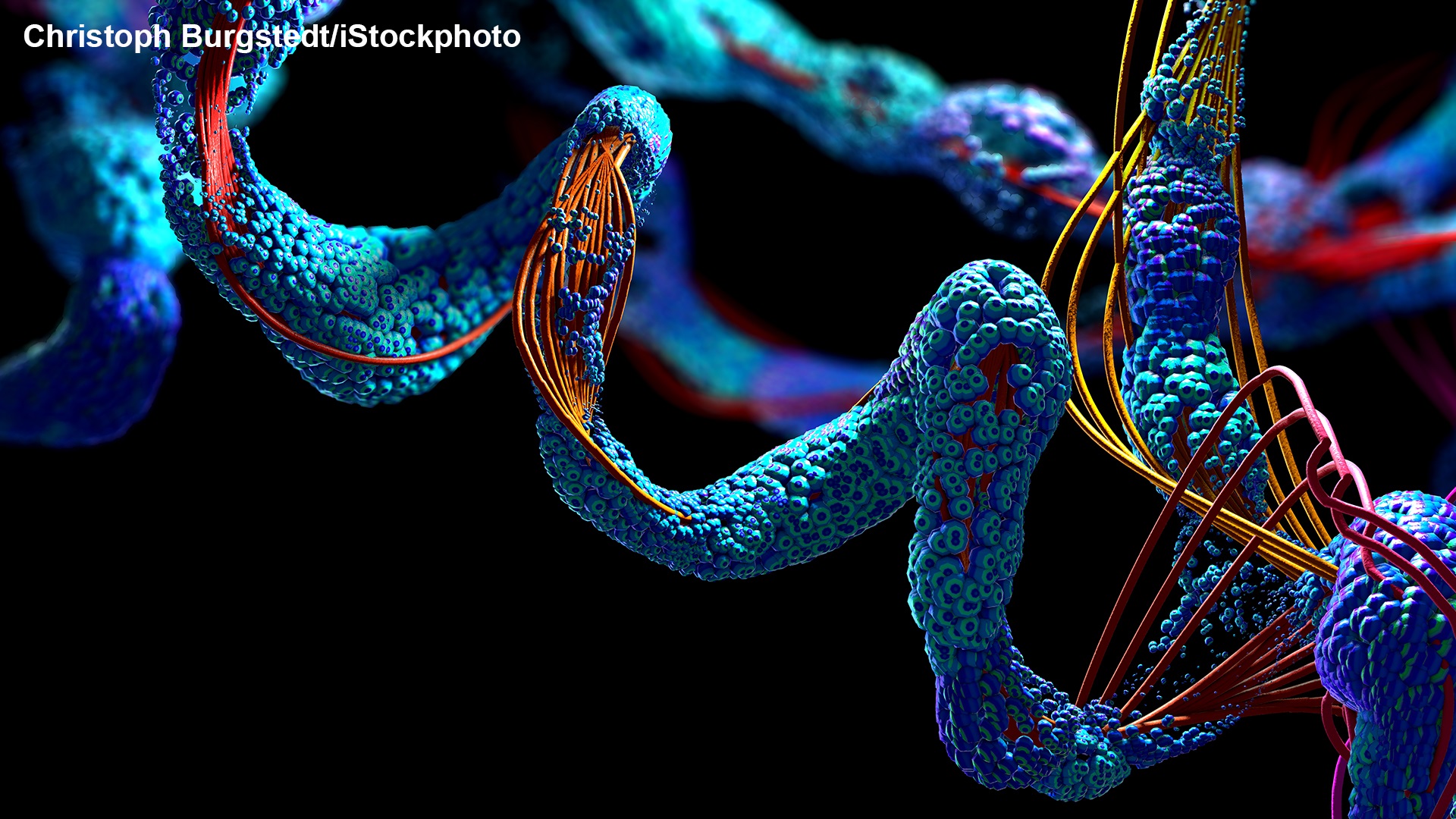
by Joachim Pietzsch
Back to Proteins
Proteins carry their importance within their name. It is derived from the Greek word “proteios”, which means “standing in front” or “in the lead”, and has been given to them by the Swedish chemist Jöns Jacob Berzelius, then “the elder statesman of the world of chemistry”, on July 10th, 1838. In a letter to his young Dutch colleague Gerrit Mulder he wrote on that day: “Le nom protéine que je vous propose pour l’oxyde organique de la fibrine et de l’albumine, je voulais le deriver de πρωτεῖος, parce qu’il parait etre la substance primitive ou principale de la nutrition animale.“[1]
The research on proteins made great progress during the next hundred years. Their nature as macromolecules, composed by amino acids that are tied together by peptide bonds, was revealed, and many of their physiological functions, which went far beyond the nutritional aspects Berzelius mentioned, were discovered. Proteins proved to possess an extraordinary structural complexity and variety. Most scientists were therefore convinced that only proteins would be able to carry the hereditary information of genes. It came as a great surprise when Oswald T. Avery found out in 1943 that genes were not made up of proteins but of the nucleic acid DNA – and thus opened the race for the structural determination of genes.
For almost half a century then, from the elucidation of the structure of DNA by Watson and Crick and the publication of their seminal paper in Nature on April 25, 1953, until the publication of the working draft of the human genome in Nature and Science on February 15 and 16, 2001, respectively, genes seemed to be the most important molecules of life, at least in the public perception. In fact, they never were, as scientists always knew even if they tended to overemphasize the importance of genes themselves. After the human genome had been deciphered, the attention of science shifted „Back to proteins“, as Johann Deisenhofer (Nobel Prize in Chemistry 1988) titled his talk at the Lindau Nobel Laureate Meeting in 2002, in which he clearly stated:
„Obviously proteins are an essential ingredient of cells. Genes alone can do nothing. Life comes with proteins.“
(00:03:02 - 00:03:18)
“Proteins can do Almost Everything”
Every modern textbook of biology acknowledges the importance of proteins. „When we look at a cell through a microscope (...) we are, in essence, observing proteins. Proteins constitute most of a cell’s dry mass. They are not only the cell’s building blocks; they also execute nearly all the cell’s functions (...) Before we can hope to understand how genes work, how muscles contract, how nerves conduct electricity, how embryos develop, or how our bodies function, we must attain a deep understanding of proteins.“[2] Far-sighted as he was, Francis Crick (Nobel Prize in Physiology or Medicine 1962) had formulated similar thoughts already in 1958 in his paper „On Protein Synthesis“[3]: „It is an essential feature of my argument that in biology proteins are uniquely important (…) Watson said to me, a few years ago, ‘The most significant thing about the nucleic acids is that we don’t know what they do.’ By contrast the most significant thing about proteins is that they can do almost everything (…) Once the unique role of proteins is admitted there seems little point in genes doing anything else (…) In the protein molecule Nature has devised a unique instrument in which an underlying simplicity is used to express great subtlety and versatility; it is impossible to see molecular biology in proper perspective until this peculiar combination of virtues has been clearly grasped.“ „On Protein Synthesis“ is still worth reading, although it was published some years before Crick and others deciphered the genetic code. It also contains the first version of what became known as the „central dogma of molecular biology“, popularly spelt: DNA makes RNA makes proteins. What a pity that Francis Crick never lectured in Lindau! In fact, he visited the Nobel Laureate Meeting once, together with James Watson in 1981, but did not give a talk on this occasion. Many other laureates, however, who have substantially contributed to protein research, lectured in Lindau during the last decades. Their talks allow us to a have a closer look at the cellular life of proteins, from their birth in the ribosome to their death in the proteasome.
Born in the Ribosome
While the doors to the structural elucidation of genes and proteins were opened in Cambridge in England by means of X-ray crystallography, the door to the cell was opened at the Rockefeller Institute in New York by means of electron microscopy by Albert Claude, Christian de Duve and George Palade who jointly received the Nobel Prize in Physiology or Medicine in 1974 for their discoveries concerning "the structural and functional organization of the cell". In the mid-50s Palade had discovered the birthplace of proteins, small granular components in the cytosol that he initially dubbed microsomes and that we know today as ribosomes (a name RB Roberts suggested in 1958). While Palade (in whose lab Günter Blobel, winner of the Nobel Prize in Physiology or Medicine 1999, worked as a post-doc) never came to Lindau, his two Co-Laureates did, de Duve six times and Albert Claude once in 1975. He repeated his Nobel lecture, and gave a personal account of his life in research and an appraisal of cell biology:
(00:25:18 - 00:27:19)
„At about the same time, with the help of electron microscopy, the microsomes became the endoplasmic reticulum. Looking back 25 years later, what I may say is that the facts have been far better than the dreams. In the long course of cell life on this earth it remained, for our age for our generation, to receive the full ownership of our inheritance. We have entered the cell, the Mansion of our birth, and started the inventory of our acquired wealth. For over two billion years, through the apparent fancy of her endless differentiations and metamorphosis the Cell, as regards its basic physiological mechanisms, has remained one and the same. It is life itself, and our true and distant ancestor. It is hardly more than a century since we first learned of the existence of the cell: this autonomous and all-contained unit of living matter, which has acquired the knowledge and the power to reproduce; the capacity to store, transform and utilize energy, and the capacity to accomplish physical works and to manufacture practically unlimited kinds of products.”
All of these tasks Claude mentions are primarily conducted by proteins. The microsomes, located alongside the endoplasmatic reticulum, turned out to be „the universal factories that produce proteins continuously“ as Ada Yonath (Nobel Prize in Chemistry 2009) reminded her audience with an illustration from a children’ book in her Lindau lecture „The Amazing Ribosome“ in 2010.
(00:07:22 - 00:08:01)
Yonath has been the pioneer in the scientific adventure of elucidating the structure of the ribosome. She embarked on it already at the end of the seventies when the scientific community assumed this to be impossible because of the sheer size and complexity of the ribosome molecule. Yet after more than two decades of intense research, Yonath succeeded and in 2000 published, in parallel with and independently from her co-laureates Ramakrishnan and Steitz, the structure of the ribosome in atomic resolution. This lead to a precise understanding of its function and of the birth of proteins, as Yonath nicely explained and visualized in her 2010 lecture:
(00:11:15 - 00:16:24)
From Chain to Function
Proteins are born in the ribosome as a one-dimensional chain. To become functional, they have to fold up into a three-dimensional structure. The first three-dimensional structure of a protein was determined with the help of X-ray crystallography by John Kendrew in 1958. This protein was myoglobin, the molecule that stores and releases oxygen in the muscle. It contains about 2,600 atoms, and Kendrew had to analyze about 250,000 X-ray diffractions from 110 myoglobin crystals to reach his goal. In January 1946, Kendrew had joined the group of Max Perutz at the Cavendish laboratory in Cambridge. Inspired by J.D. Bernal and under the leadership of Sir Lawrence Bragg, Perutz had begun to tackle a more difficult problem already in 1937: To solve the structure of hemoglobin, the oxygen carrier of the blood and four times as large as myoglobin. As a pioneer in his field who dealt with a huge molecule and had to overcome many methodological problems, it took Perutz more than two decades to succeed, and so he became the runner-up to Kendrew. Both shared the Nobel Prize in Chemistry in 1962. While Max Perutz participated in the Lindau Nobel Laureate Meetings three times between 1986 and 1999, John Kendrew attended the meeting once, already two years after he had received the Nobel Prize. He gave a brilliant state-of-the-art lecture on „Recent Studies of the Structure of Proteins“. After modestly stating that he “had been studying a simpler protein” than Perutz, he shared insights on both the myoglobin and the hemoglobin structure with his audience, expressed his hope that both structures would help to better understand the function of proteins and then extensively discussed the relationship between the sequence and the three-dimensional structure of proteins.
(00:31:48 - 00:37:31)
The hypothesis Kendrew refers to at the end of this cutting, stems from the work of Christian Anfinsen. In investigations on the enzyme ribonuclease in the 1950s, Anfinsen demonstrated that all information needed to fold up a protein is encoded within its amino acid sequence. No further genetic information than that found in DNA is needed to form a three-dimensional protein. The driving force of correct protein folding is the tendency of each system to assume a state of minimum energy. The native state of a protein represents the thermodynamically most stable conformation in the intracellular environment, and it reaches this state via a funnel of decreasing energy. Nevertheless, it remains a remarkable paradox, as Cyrus Levinthal noted in 1969, that a protein folds within less than a second while it theoretically would need many billion years to try out all its conformational possibilities. A relatively small protein with 100 amino acids and one rotatable bond per residue with two favored orientations, for example, has two to the power of 100 possibilities to fold. Even if it checked 100 billion different conformations per second, it would need 100 billion years to probe all of them. While a lot of progress has been made in understanding the mechanisms of protein folding and the connections between incorrect folding and disease, it is still not possible today to predict the three-dimensional structure of a protein from its amino acid sequence. The situation appears to be even more complicated because we know today a multitude of post-translational modifications, which can influence the structure of proteins and thereby modulate their activity considerably.
Christian Anfinsen participated in the Lindau Nobel Laureate Meetings six times. In his talk „Peptide Synthesis - a Useful Tool in the Study of Protein Structures and Function“ he briefly looked back on the work that had earned him a Nobel Prize in Chemistry in 1972:
(00:03:06 - 00:04:38)
A Decisive Molecular Switch
The interaction of folded proteins needs to be constantly controlled and regulated to ensure that a cell functions properly. The activity of proteins can be switched on or off by adding or subtracting phosphate. This reversible protein phosphorylation arguably is the most important mechanism of cellular regulation. It was discovered by Edmond Fischer and Edwin Krebs, when they studied glycogen phosphorylase. The role of this enzyme had been identified by Carl and Gerti Cori (Nobel Prize in Physiology or Medicine 1947) in the 1930s: It catalyzes the first step in the degradation of glycogen in the muscle and the liver, a reaction that needs to be turned on very quickly if energy is needed. Krebs had been a student of the Cori’s in St. Louis, and Fischer had been a student of Kurt H. Meyer, a European pioneer of enzymology, in Geneva. Both met at the University of Washington in Seattle in the mid-50s, shared a lab, and decided to have a crack at a problem the Coris had raised but later abandoned: Does glycogen phosphorylase need adenosine monophosphate (AMP) as a co-factor to get activated? While the general role of AMP in the allosteric regulation of enzymes was elucidated some years later by Jacques Monod (Nobel Prize in Physiology or Medicine 1965), Fischer and Krebs discovered the covalent regulation of enzymes. Glycogen phosphorylase gets activated when covalently binding a phosphate, with the catalytic help of a specialized enzyme, a kinase. The fundamental importance of this discovery was not immediately apparent, so that Fischer and Krebs had to wait some decades before they received their well deserved Nobel Prize in Physiology or Medicine in 1992. In the meantime, it had become clear that phosphorylating kinases and dephosphorylating phosphates are abundant in living cells to steer intricate networks of intracellular communication by amplifying, fine-tuning and integrating signals, influencing many aspects of cellular behavior from glucose mobilization to oncogenesis. More than one third of the many thousands different proteins, which are active in an average mammalian cell, are phosphorylated at any given time.[4] Edmond Fischer has been an almost regular participant of the Lindau Meetings since he received the Nobel Prize. He first came to Lindau in 1993 and gave a detailed account of his discovery in his lecture „Cellular Regulation by Protein Phosphorylation“. In 2003, he presented his field of research in the broader context of „How Proteins Communicate with one Another to Integrate Cellular Signals”, emphasizing the importance of understanding the proteome:
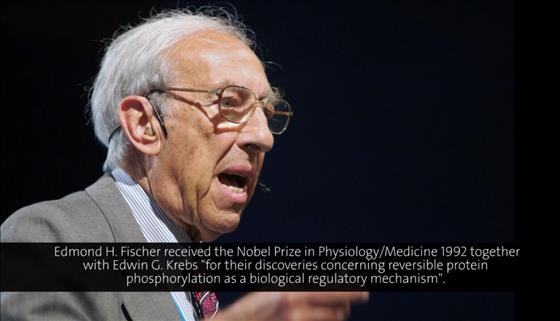
(00:04:10 - 00:06:10)
The Protein Universe
Not all proteins fulfill their tasks within the cytoplasma. Many proteins involved in vital biological functions, such as the transport of nutrients into cells or nerve impulses, span the fatty membranes that surround every cell. Buried within the membrane as they are, these important proteins were long thought to be impossible to access and crystallize for structural studies.
The seemingly impossible was at last achieved by Johann Deisenhofer, Robert Huber and Hartmut Michel in 1986, for which they received the Nobel Prize in Chemistry in 1988. Their breakthrough was all the more important as their target had been the photosynthetic reaction center of a bacterium, and photosynthesis surely is the most important chemical reaction on earth, being the prerequisite of life.
All three Laureates are faithful participants of the Lindau Meetings, where they regularly teach hundreds of young scientists from all over the world to appreciate the achievements and possibilities of protein research. It is interesting to watch Robert Huber explain to the younger generation why it is worth studying proteins in his 2008 lecture on the „Beauty and Usefulness of the Building Blocks of Life: The Architecture of Proteins“, where he also mentions the three major techniques applied in structural studies: electron microscopy, X-ray crystallography and nuclear magnetic resonance (NMR).
(00:01:32 - 00:05:36)
NMR is a technique based on discoveries by Otto Stern and Isidor Isaac Rabi (Nobel Prize in Physics in 1943 and 1944, respectively). As an analytical tool it was in principle developed by Felix Bloch and Edward Purcell (Nobel Prize in Physics 1952) and subsequently brought to fruition as a high resolution methodology by Richard Ernst (Nobel Prize in Chemistry in 1991). The application of NMR to proteins and other biological macromolecules was first put into practice by Kurt Wüthrich, an achievement that earned him the Nobel Prize in Chemistry in 2002. In contrast to X-ray crystallography, NMR allows to study proteins in solution, in an enviroment, which is similar to that in a living cell – an advantage of considerable importance. With deep knowledge and a good sense of humor, Kurt Wüthrich reminded his audience in Lindau in 2012 of the importance of proteins – in his lecture „Structural Genomics – Exploring the protein universe“.
(00:03:52 - 00:09:55)
Vital Degradation
None of our proteins lives as long as we do. Today, this seems to be a trivial remark. The notion that proteins are subject to a constant turnover, however, is hardly more than 70 years old. Before that time, structural and functional proteins were regarded as relatively stable constituents of our body, endangered solely by minor “wear and tear” forces, with the exception of dietary proteins whose task was seen in serving as an energy-providing fuel. This “combustion engine concept” was first challenged by Rudolf Schönheimer in 1941 when he suggested in his Edward K. Dunham Lecture at Harvard University that „not only the fuel, but the structural materials are in a steady state of flux. The classical picture must thus be replaced by one which takes account of the dynamic state of body structure“.[5]
Yet even between the “1950s and 1980s, scientists were focusing mostly on how the genetic code is transcribed to RNA and translated to proteins, but how proteins are degraded has remained a neglected research area”, Aaron Ciechanover stated in his Nobel Lecture in 2004. Together with his doctoral father Avram Hershko and with Irwin Rose he earned the Nobel Prize in Chemistry because they had discovered the central mechanism of protein degradation in the cell: the ubiquitin-proteasome pathway. Since 2005, Ciechanover has participated in almost every Nobel Laureate Meeting in Lindau.
In clear words he explains, why protein degradation is so important
(00:05:24 - 00:07:41)
and elegantly illustrates how the ubiquitin system functions
(00:22:42 - 00:25:30)
Time and again, it becomes very obvious in Ciechanover’s lectures that, as he puts it, our proteins have to die so that we shall live.
[1] Both quotes from Tanford C, Reynolds J. Nature’s robots. A history of proteins. Oxford University Press, 2001, p. 11ff.
[2] B. Alberts et al., Molecular Biology of the Cell, Fifth Edition, 2008, Garland Science,
New York, USA, p. 125
[3] Crick, Francis. "On Protein Synthesis." Symposia of the Society for Experimental Biology 12, (1958): 138-163, p. 139
[4] B. Alberts et al., Molecular Biology of the Cell, Fifth Edition, 2008, Garland Science,
New York, USA, p. 176
[5] Schoenheimer, R. The Dynamic State of Body Constituents (1942). Harvard University Press, Cambridge, Massachusetts, USA, quoted from: Ciechanover A. Intracellular protein degradation (...) (Nobel lecture), http://www.nobelprize.org/nobel_prizes/chemistry/laureates/2004/ciechanover-lecture.html, p. 152
Additional Lectures by the Nobel Laureates associated with Life of Proteins:
Introductory Mini Lecture on "Life of Proteins".
James Watson (1967): RNA Viruses and Protein Synthesis.
Lawrence Bragg (1968): History of the Determination of Protein Structure.
Hugo Theorell (1969): Enzyme Research Earlier and Today.
Dorothy Hodgkin (1970): Structure of Insulin.
Richard Synge (1970): Proteins and Poisons in Plants.
Hartmut Michel (1998): From Photosynthesis to Respiration: Structure and Function of Energy Transforming Membrane Complexes.
Roderick McKinnon (2005): Ion Channels – Life’s Electronic Hardware.
Edmond Fischer (2007): Protein Crosstalk in Cell Signaling.
Robert Huber (2007): Proteolyis and its Regulation, a Molecular Basis.
Martin Chalfie (2009): GFP and After.