(Small) Molecules of Life
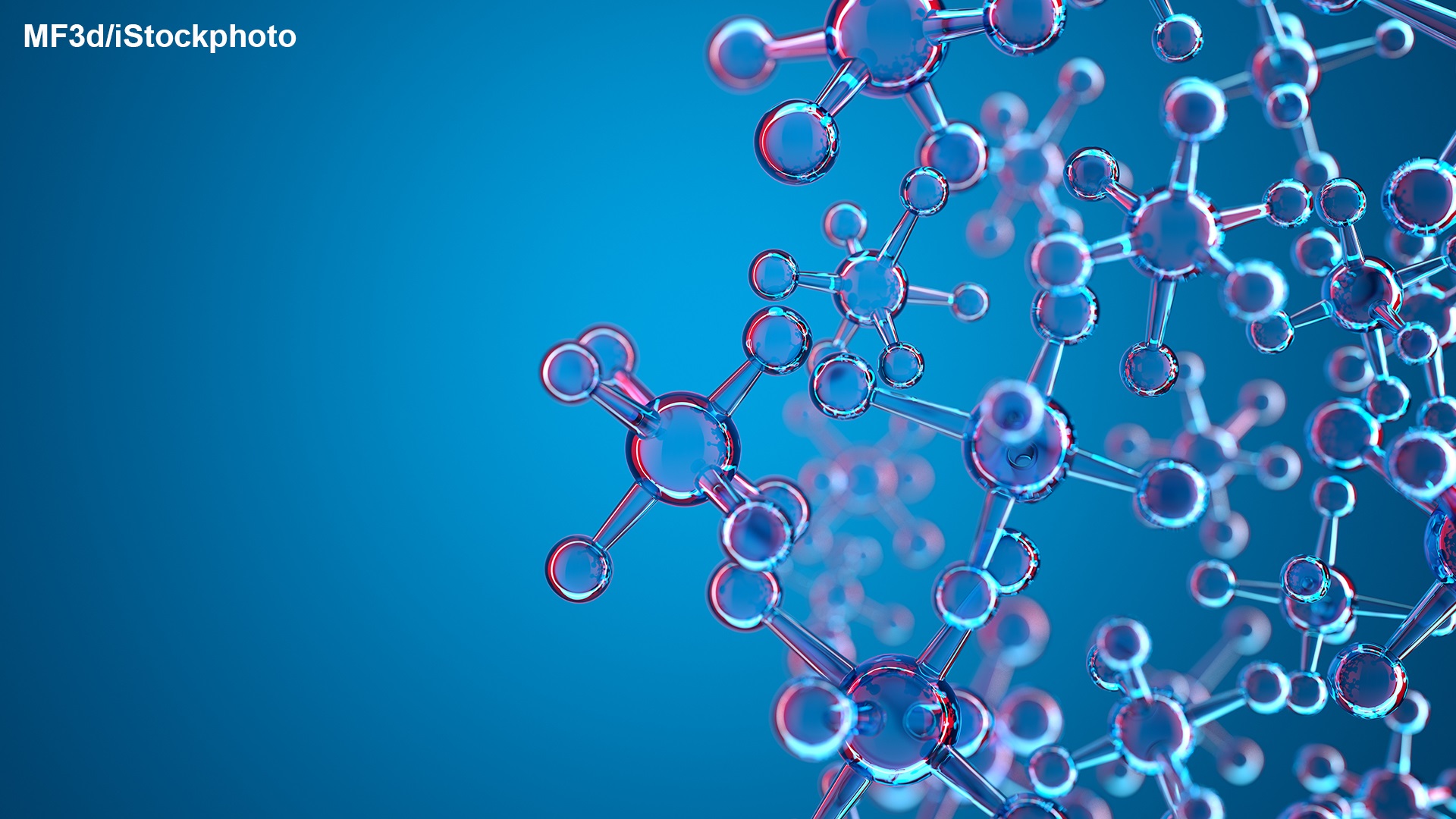
by David Siegel
An Economic View on Human Biochemistry
“The human body’s chemical value is just about a couple of bucks.” - a frequently read line and a great understatement. While it is indeed true that the value of the chemical elements found in a human body, mainly oxygen, carbon, hydrogen, nitrogen, calcium and phosphorus in their pure form is not more than a couple of dollars or euros, this is not what we are actually made of. Our bodies are rather incredibly rich agglomerations of biomolecules, made from those elements, ranging from simple compounds like water to huge biopolymers like DNA or collagen, the major structural protein in our skin, hair, muscles or tendons. In this chemical treasure trove the pure elements are barely represented - neither by mass nor by number.
Biomolecules, as well as their mixtures and structures (think of organs, eggs, bone-marrow or blood) fulfil key functions in human biology and thus gain their worth. A fact which needs to be acknowledged when attempting the calculation of a body’s “chemical value”. It is obvious that such calculations are sketchy as human body parts are usually not sold freely - at least not on legal marketplaces. Still, the magazine Wired attempted an estimate in its February 2011 issue [1] and arrived at several million US dollars for the components of a human body, sold individually.
The difference between the two prices, “a couple of bucks” and several million dollars, one for the plain elements, the other for molecules and structures thereof, is a (sketchy) measure of the value of chemical information inherent to our bodies’ biomolecules, which is, again, a measure for the incredible degree of optimization achieved by evolutionary processes. In his 1977 Lindau lecture, German Nobel Laureate Manfred Eigen gave the audience a straightforward yet impressive introduction on the power of evolution with respect to the generation of such (useful) biochemical information:
(00:01:53 - 00:04:48)
Structuring the Biochemical Treasure Trove
The molecular information generated in several billion years of evolution is vast. Even today, no one would dare to claim to understand all the chemistry going on in a human body – even keeping track of the tens of thousands of molecules we do know about proves difficult enough. Still, there are ways of classifying our biomolecules which can make life a lot easier.
First off, there are the biopolymers, chain-like molecules built from recurring, well-defined links. Our DNA is a biopolymer built from a set of four nucleobases. Similarly, our proteins are biopolymers built from 21 amino acids. Biopolymers are characterized by an easily comprehensible, linear construction principle, which – at the same time – allows for a theoretically limitless number of molecules. The only restricting factor is the achievable chain length. Hence, DNA and most proteins are what biochemists consider “large” molecules.
Next to the large biopolymers, there are non-polymeric molecules most of which qualify as “small”. By convention, this means that their atomic mass is below 900 Dalton, the mass of 900 hydrogen atoms, 56 oxygen atoms or 50 water molecules. In our bodies, small molecules take over a variety of functions – nutrients like carbohydrates and lipids partake in metabolism and thus act as a source of energy and chemical building blocks required for growth and development. Neurotransmitters, hormones and pheromones are involved in signal transduction. And vitamins and cofactors support enzymes in conducting essential biochemical reactions, to name only the most prominent examples.
Small molecules may enter the human body via inhalation, absorption through the skin and most importantly: via food. Once taken up, they are subject to a variety of metabolic processes. Small molecule nutrients are broken down to gain energy and chemical building blocks or converted to other compounds, which can be stored or transported more efficiently. Potentially toxic substances are equally degraded or chemically modified to facilitate excretion. And, most compounds are, to a lower extent, subject to random chemical reactions, which are unintended by the body’s biochemical machinery. Taken together, these processes account for the huge number of small molecules found in our bodies. Today, we know of more than 40.000 such compounds [2]. Reasons enough to put DNA, RNA and the proteins on the side and dive into the world of our (small) molecules of life.
Dealing with Macronutrients: Central Carbon and Energy Metabolism
Next to the proteins, small molecule macronutrients like carbohydrates and lipids are essential for our survival. As opposed to micronutrients, they are required in rather large quantities and can be considered raw materials required both as “fuels” and for the generation of chemical building blocks. Our body is equipped with a highly optimized biochemical machinery, allowing for an incredibly efficient utilization of such macronutrients. Humans metabolize as much as 93 to 95 % of the macronutrients contained in a typical mixed meal [5].
In the world of macronutrients, everything revolves around carbon. To obtain energy, our body converts energy-rich, carbon-based macronutrients to energy-poor, stable molecules, most of all carbon dioxide. Details on the chemical journey of carbon, from macronutrient to carbon dioxide and the associated Nobel Prizes, can be found in the Mediatheque’s Topic Cluster on Carbon. Notably, these so-called oxidation processes do not occur in a single step but proceed through biochemical pathways like glycolysis or the citric acid cycle, involving a wide range of intermediate compounds. Taken together, these small molecules are referred to as central carbon metabolites or energy metabolites. They are found in practically every cell.
However, our bodies do not stop at just degrading macronutrients. To a certain degree, they also interconvert them. One of these interconversion processes leads to an effect dreaded by many: weight gain, i.e. the accumulation of fatty acid triglycerides in fat cells. Fat pads are in essence reservoirs of small molecules used for energy storage. In this context, it is a common misconception that the build-up of fat can be avoided by a fat-free diet. Our bodies possess the ability to biosynthesize fats from very small building blocks, containing only two carbon atoms. The body can source these building blocks from carbohydrates and proteins and thus produce fat de novo. At the 1966 Lindau meeting, 1964 Nobel Laureate Feodor Lynen, who had been honoured together with Konrad Bloch for his contributions to the elucidation of cholesterol and fatty acid metabolism, explained to the audience why humans are largely independent of dietary fat:
(00:00:15 - 00:04:11)
Macronutrients vs. Micronutrients
Towards the end, Lynen mentions that certain fatty acids cannot be biosynthesized and hence need to be taken up with the diet in quantities comparable to those applicable to vitamins. These two fatty acids, α-linolenic acid and linoleic acid, belong to the group of micronutrients. Micronutrients are essential, but not required for energy generation. They rather support vital metabolic reactions, either directly, as enzyme cofactors, or indirectly, as building blocks for other biomolecules.
Minerals, trace elements (e.g. iodine) and vitamins complement the spectrum of micronutrients. In the realm of the Nobel Prizes, vitamins in particular play an outstanding role. No other group of small molecules has motivated more Nobel Prizes. This may be explained by considering that the concept of micronutrients in general and vitamins in particular was not well established until the first half of the 20th century. The story of vitamin discovery hence coincides with the story of the Nobel Prize, which was first awarded in 1901. Whereas (with few exceptions) the major macronutrients were isolated before Nobel Prizes were awarded, vitamin researchers just were in the right spot at the right time.
Vitamins
Discovery and Nobel Prizes
All 13 vitamins we know of today (A, B1, B2, B3, B5, B6, B7, B9, B12, C, D, E, K) were discovered in a period of about three decades, from 1913 to 1941. With the Nobel Prizes being awarded since 1901, the chances of receiving an award for vitamin research could not have been better and, in fact, as many as eight Nobel Laureates owe their Prizes directly to work on the discovery, isolation or structural characterization of novel vitamins [3]. Several additional Prizes were given for work involving vitamins in part.
However, it was only in 1929 when the Karolinska Institutet decided to honour vitamin researchers. After all, the concept of micronutrients and vitamins was still new, even the term “vitamin” had only been coined in 1912 (based on the false assumption that all vitamins feature an amine group, vitamin was short for “vital amines”). And by no means were vitamin pills available over the counter like they are today.
Still, once the importance of vitamins became apparent, a true wave of vitamin Nobel Prizes ensued. It began with the 1929 Prize in Physiology or Medicine, which went to Frederick Hopkins and Christiaan Eijkman for their work towards the discovery of the vitamins A and B1, respectively. These two vitamins were the first discovered and the first connected to a Nobel Prize. Interestingly, neither Eijkman nor Hopkins had isolated the pure vitamins or determined their structure. They merely pointed out that certain foods (milk in the case of vitamin A and brown rice in the case of vitamin B1) have the potential to cure certain conditions arising from an unbalanced diet. Others later identified the actual compounds responsible.
In 1937, both, the Prize in Physiology or Medicine and the Prize in Chemistry went to vitamin research. And both Prizes were motivated essentially by one compound: ascorbic acid or vitamin C. Albert von Szent-Györgyi Nagyrápolt was rewarded in Physiology or Medicine for its isolation, whereas Walter Haworth received one of the Chemistry Prizes for synthesizing the vitamin (in 1934). Haworth’s co-recipient, Paul Karrer, was acknowledged for his work on the vitamins A, E and the B vitamins.
Ascorbic acid, the lack of which caused the famous scurvy amongst sailors, was long sought after. It had been known for quite a while that foodstuffs like lemon juice can prevent scurvy. However, that a single small molecule compound was responsible for this effect could only be established in the 1930s. In his 1969 Lindau lecture, the Hungarian Szent-Györgyi described how he “accidentally” isolated vitamin C while wondering about why certain fruits turn brown when damaged, while others do not:
(00:30:32 - 00:37:08)
The next Nobel Prize for vitamin research followed promptly in 1938, with the German organic chemist Richard Kuhn being distinguished for his work on the structure and synthesis of the vitamins B2 and B6. Kuhn later dedicated his 1952 Lindau lecture to the one foodstuff which was at the core of the discovery of the first vitamins: milk. Since most mammals live of nothing but milk during the first part of their life, a healthy mother’s milk must contain all macronutrients and micronutrients required for growth and development. This includes all vitamins, as Kuhn points out:
(00:00:12 - 00:02:58)
Another five years later, Henrik Dam and Edward Doisy shared the 1943 Prize in Physiology or Medicine, being rewarded for the discovery and structural elucidation of vitamin K.
The Special Case of Vitamin B12
Vitamin B12 takes a special role amongst the vitamins. It is the only vitamin that contains a metal atom (cobalt), as part of its structure. With a weight of 1580 Dalton it is the heaviest of the vitamins. It is the last vitamin to be structurally characterized. And it was linked to a Nobel Prize before it was even known to exist.
This Prize was given in 1934 to George Whipple, George Minot and William Murphy “for their discoveries concerning liver therapy in cases of anaemia". The scientists had shown, that certain fatal types of anaemia, a disease caused by the lack of red blood cells, could be treated by having patients eat liver. Thousands of lives could be saved by this method. However, when the Prize was awarded, the reasons for this success were unclear and only in 1948 the actual active agent, vitamin B12, could be isolated in crystalline form.
It then took another seven years before the British biochemist Dorothy Hodgkin was able to determine the correct structure of the crystalline compound by using the emerging technique of
x-ray diffraction crystallography
. Hodgkin was awarded the 1964 Chemistry Nobel Prize, inter alia for this achievement. It was the last Nobel Prize linked to the discovery, structural elucidation or synthesis of a vitamin so far.
Vitamins: A Story with an Open End
But of course, the story of vitamins does not end here. In the years following their discovery, researchers began to work towards an understanding of the biochemical role of the individual compounds. It was found, for example, that vitamin A is contained it the retina and directly involved in the visual process. This insight led to the 1967 Nobel Prize in Physiology or Medicine, which was given to Ragnar Granit, Haldan Hartline and George Wald "for their discoveries concerning the primary physiological and chemical visual processes in the eye".
Besides such fundamental work, numerous studies were concerned with determining the potential of vitamins in preventing or curing mental and physical diseases. Unfortunately, not all of these studies were conclusive and by no means is our knowledge on the biochemical functions or health effects of vitamins complete. It is thus not surprising that the wellknown recommended daily allowance (RDA) or dietary reference intake (DRI) are, in part, subject to heavy discussion.
Linus Pauling and Vitamin C
One of the most prominent critics of these RDA values was double Nobel Laureate Linus Pauling. Pauling claimed that vitamin C megadoses (around 10 gram per day, or 12500 % of the current EU-RDA) would be suited to treat cancer and support health in general. He himself took at least 10 gram of vitamin C per day for more than 20 years. In his 1981 Lindau lecture, Pauling explained how he conceived his 10 g/day recommendation:
(00:09:45 - 00:15:18)
To date (2013), it has not been proven that vitamin C megadoses are suited as a cancer therapy and the studies Pauling describes in his talk have been shown to contain systematic flaws. The Linus Pauling Institute at the Oregon State University, founded in 1973 by Pauling and colleagues, now distances itself from the claim that vitamin C is effective in cancer therapy and recommends a rather low daily intake of 400 milligram (or 500 % of the current EU-RDA) based on the “currently available epidemiological, biochemical, and clinical evidence” [4].
Signalling: Neurotransmitters, Hormones and the Sense of Smell
Leaving behind nutrition and metabolism, we arrive at another important biochemical domain of small molecules: the transduction of signals. Just as metabolic processes, chemical signalling is a pillar of life. It perpetually occurs on almost all scales – inside individual cells or in-between different cells of the same organism, between an individual organism and the environment, but also between organisms, be they of the same or different species.
Hundreds of messenger molecules, belonging to all kinds of compound classes are known to act in our bodies. However, whenever a signal has to be transmitted very rapidly or over a very long distance, the odds are that a small molecule is involved. This is due to several reasons: small molecules can be made and processed much faster than proteins, they can, in part, migrate through cell walls and, in the case of smells or pheromones, be distributed via air.
Neurotransmission
A classic example of rapid chemical signalling is neurotransmission. Today we know, that signal transduction between neurons is achieved by small molecule neurotransmitters such as glutamate, GABA, glycine, dopamine or serotonin. However, for a long time the crucial role of these small molecules was not realized and neurotransmission was assumed to be merely electrical in nature. Only in 1920, the pharmacologist Otto Loewi, then at the University of Graz in Austria, showed with a famous experiment that a chemical substance must be involved. Loewi himself described this experiment as follows [6]:
“The hearts of two frogs were isolated, the first with its nerves, the second without. Both hearts were attached to Straub canulas filled with a little Ringer solution. The vagus nerve of the first heart was stimulated for a few minutes. Then the Ringer solution that had been in the first heart during the stimulation of the vagus was transferred to the second heart. It slowed and its beats diminished just as if its vagus had been stimulated. Similarly, when the accelerator nerve was stimulated and the Ringer from this period transferred, the second heart speeded up and its beats increased. These results unequivocally proved that the nerves do not influence the heart directly but liberate from their terminals specific chemical substances which, in their turn, cause the well-known modifications of the function of the heart characteristic of the stimulation of its nerves.”
Together with the Brit Henry Dale, Loewi then went on to discover the first neurotransmitter, acetylcholine. Both scientists shared the 1936 Nobel Prize in Physiology or Medicine. Loewi also showed that after neurotransmission acetylcholine is broken down to two other small molecules, acetate and choline, by an enzyme called acetylcholine esterase. This is one of the fastest enzymatic reactions known. A single molecule of acetylcholine esterase, which is considered to be an “evolutionary perfect” enzyme [7], can hydrolyse around 16.000 molecules of acetylcholine per second [8]. Proteins cannot be made or degraded at similar rates.
Loewi was a pioneer of neuroscience and many others followed in his footsteps. At the 2011 Lindau Nobel Laureate Meeting, 1991 Nobel Laureate Erwin Neher, who had received a shared Prize for his work on ion channels, summarized the history and current knowledge on neurotransmission:
(00:00:30 - 00:08:03)
Hormones and Related Messengers
Neurotransmission is a fast process and usually occurs at synapses linking two neurons. If cells require to communicate over longer distance, for example between different parts of the body, the may do so by means of a different group of signalling molecules, the hormones.
In his 2007 Lindau lecture, 1998 Nobel Laureate Ferid Murad familiarized the audience with intercellular, hormonal signalling. He had received the Prize together with Robert Furchgott and Louis Ignarro for the discovery of one of the smallest signalling molecules known: nitric oxide, a molecule consisting of only a nitrogen and an oxygen atom.
(00:13:34 - 00:17:23)
As for the vitamins, the discovery of hormones began not earlier than 1902, making hormone research “highly susceptible” to Nobel Prizes. However, while in that year, the Brits Wiliam Bayliss and Ernest Starling did discover secretin, the first hormone ever identified, and while the two scientists even coined the term “hormone” in 1905, they never received the Nobel Prize.
Secretin is not a small, but a “large” protein molecule. It is released from the small intestine upon the arrival of food from the stomach. The hormone then triggers the release of digestive juices by the pancreas, a classical example of between-organ signalling. And, importantly, a process not involving the brain, as previously assumed. Curiously, Ivan Pavlov, introduced as the father of chemical signalling by Ferid Murad above, received the unshared 1904 Nobel Prize in Physiology or Medicine for a theory of digestion, which still put the brain at the centre of the physiology of gastric secretion. The award was given, although Pavlov’s theory had been proven incorrect by Bayliss and Starling two years before. A controversial decision.
Another “large” protein hormone tied to a much discussed Nobel Prize is insulin. The story of its discovery, and a description of the controversy around the associated 1923 Nobel Prize in Physiology or Medicine, may be found on the website of the Nobel Foundation [10].
In 1939, the first Nobel Prize involving small molecule hormones was given to the German Adolf Butenandt. The chemist received half of the award for his work on sex hormones. And it was hard work indeed. Butenandt and his team had processed thousands of litres of urine, collected from pregnant women, in order to isolate a couple of milligrams of the sex hormone oestrone. Butenandt also isolated androsterone from men’s urine and progesterone from pig ovaries, determining the structure of the compounds. Jointly with his co-recipient, the Swiss-Croatian Leopold Ruzicka, he eventually established that several sex hormones may be synthetically derived from a common precursor, cholesterol, the first known steroid.
In 1950, three scientists received the Nobel Prize for Physiology or Medicine for a similar feat. Edward Kendall and Philip Hench, both from the USA, and the Swiss-Polish Tadeus Reichstein were rewarded for their work on the hormones of the adrenal cortex. It included the isolation and structural characterization of about 29 small molecules found in the adrenal cortex and some early studies on their biological effects. The best known of these compounds is cortisone, which was later shown by Hench to be an effective anti – rheumatic agent. Like the sex hormones, the hormones of the adrenal cortex belong to the chemical group of steroids. At the 1954 Lindau Nobel Laureate Meeting, Reichstein told the story of their discovery:
(00:00:15 - 00:07:29)
It may be added that, in 1934, Reichstein also developed a technical procedure for the large-scale production of vitamin C. The so-called Reichstein process relies on the use of the synthetic power of bacteria and has dominated industrial vitamin C production for decades. It is still used today in modified form [9].
Back in the world of hormones, the next Nobel Prize was awarded in 1971 to Earl Sutherland "for his discoveries concerning the mechanisms of the action of hormones". Sutherland had elucidated the concept of first and second messengers (introduced by Ferid Murad above) and discovered the important (small) second messenger molecule cyclic AMP. Unfortunately, Sutherland never came to Lindau. Six years after his distinction, Roger Guillemin and Andrew V. Schally shared one of the 1977 Nobel Prizes in Physiology of Medicine "for their discoveries concerning the peptide hormone production of the brain". They had investigated how the brain controls certain hormone producing glands, like the thyroid, and found that it uses yet another set of “large” protein hormones to do so.
The next Nobel Prize for small molecule signalling came in 1982 and was awarded jointly to Sune Bergström, Bengt Samuelsson and John Vane "for their discoveries concerning prostaglandins and related biologically active substances". Prostaglandins are locally acting messenger molecules affecting only those cells in the vicinity of the signalling cell (hence, they formally do not belong to the class of hormones). They exert an extremely wide variety of strong physiological effects and act in practically every organ, tissue, and cell of our bodies [11]. Prostaglandins trigger the constriction and relaxation of muscle tissue, induce labour and play important roles in pain, fever and inflammation, to name only a few examples. Drugs suppressing prostaglandin biosynthesis, such as aspirin or ibuprofen, are commonly used as anti-inflammatory agents, while the prostaglandins themselves have found several clinical applications including the induction of childbirth or early abortions [11].
This brings us to the last Nobel Prize given for the discovery of a small signalling molecule so far. It was given to Robert Furchgott, Louis Ignarro and Murad, who had elucidated nitric oxide signalling. Murad, who introduced this subsection, told the story of nitric oxide later in his talk:
(00:27:50 - 00:30:58)
Signalling Beyond Body Borders
After all, chemical signalling does not end at the borders of our bodies. We routinely send and receive chemical signals to and from the environment. Amongst our five senses, the sense of smell and taste are charged with the reception and processing of such signals. Even though the human sense of smell does not belong to the best developed amongst mammals, its power is still impressive. Humans are able to recognize around 10.000 different odours [11]. These may convey a variety of signals – they may warn us of immediate dangers, such as fires, tell us about the quality of food or, as for the so-called social odours, inform us about sex, health, reproductive status or competitive ability of another human [12].
Curiously, the 10.000 odours we can perceive are composed from merely less than 1.000 different small molecule odorants. The number of odours can be so much higher than the number of odorants because odours are characteristic mixtures of several types of odorant molecules. The latter are typically “very small” with a mass of less than 300 Dalton. Molecules of this size are often volatile, an essential prerequisite for perception by the olfactory system.
The question, how exactly the sense of smell is able to differentiate so efficiently between so many odours has long puzzled scientists. Today we know, that the human olfactory system uses a limited range of odorant receptor types to characterize a given odour. Each of these receptors can, to varying degrees, be activated by several odorants. The odorants of a certain characteristic scent thus create a unique combinatorial pattern, which can be recognized and remembered by the brain. The two scientists who unravelled the workings of the olfactory system, Richard Axel and Linda Buck, were honoured with the Nobel Prize in Physiology or Medicine in 2004. Amongst other things they could point out which receptors are activated by which small molecule odorants. They further showed that pheromones, chemical signals directly triggering social responses, are recognized by similar receptors as smells.
In the case of pheromones, the sensitivity of the involved receptors is truly stunning. The first one to point this out was 1939 Nobel Laureate Adolf Butenandt, introduced above as the discoverer of the sex hormones oestrone, androsterone and progesterone. After receiving the Nobel Prize, Butenandt attempted the first-ever isolation of a pheromone, bombykol. It took his lab 20 years to isolate 15 milligrams of bombykol from 500.000 female silk moths. One reason for the slow success was that the female silk moth requires merely tiny amounts of bombykol to attract males. In fact, a couple of molecules suffice to trigger a reaction. Even today, this stunning sensitivity is unmatched by any form of modern analytical instrumentation. At the 1960 Lindau meeting, shortly after the discovery of bombykol, Butenandt told the audience about his experiments with the first pheromone discovered:
(00:47:03 - 01:03:36)
Conclusion
With the pheromones we conclude our excursion through the world of the small molecules of life. Clearly, the important examples of small molecules discussed here represent merely a glimpse on the vast world of biologically relevant small compounds. Some important groups of small molecules, which are not endogenous in humans, had to be by-passed for the sake of brevity. Those include toxins, medicines and drugs. Nobel Prizes related to such substances were, for example, given in 1945 and 1952 for the discovery of antibiotics penicillin and streptomycin, respectively.
Today, small molecules are rather easy to isolate and characterize, especially in contrast to proteins. In addition, sophisticated synthetic methods in many cases allow for their cost-efficient, large-scale synthesis. Beginning in the second half of the 20th century, Nobel Prizes given for the discovery of “new” small molecules have thus become rather scarce. Instead, the elucidation of entire biomolecular mechanisms, often involving a set of already known small molecules, has moved into focus. The 2004 Nobel Prize to Richard Axel and Linda Buck is a good example of that.
Still, it can be stated without doubt that we do not know all biologically relevant small molecules. The future might thus well hold some exciting surprises. And, coming back to the initial theme, small molecules do significantly contribute to the “chemical worth” of a body. As an average human, you make around 70 kilogram of adenosine triphosphate (ATP) per day [13]. If you would purify and sell only a tenth of that, it would instantly make you a millionaire.
Footnotes:
[1]http://www.wired.com/magazine/2011/01/ff_redmarkets/
[2]D.S. Wishart et al., Nucleic Acids Research 2013, 41(D1), D801-7.
[3]Carpenter, KJ, The Nobel Prize and the Discovery of Vitamins, http://www.nobelprize.org/nobel_prizes/medicine/articles/carpenter/
[4]http://lpi.oregonstate.edu/infocenter/paulingrec.html
[5]F.X. Pisunyer, Critical Reviews in Food Science and Nutrition 33 (1993) 359.
[6]Loewi, O. (1960-1961) Perspect. Biol. Med. 4, 3-25
[7]D.M. Quinn, Chemical Reviews 87 (1987) 955.
[8]T.L. Rosenberry, Advances in Enzymology and Related Areas of Molecular
Biology 43 (1975) 103.
[9]C. Bremus et al., Journal of Biotechnology 124 (2006) 196.
[10]http://www.nobelprize.org/educational/medicine/insulin/discovery-insulin.html
[11]http://www.nobelprize.org/nobel_prizes/medicine/laureates/2004/press.html
[12]P.A. Brennan et al., Philosophical Transactions of the Royal Society
B-Biological Sciences 361 (2006) 2061.
[13]S.E. DiCarlo and H.L. Collins, Advances in Physiology Education 25 (2001) 70.