The Rise of Life
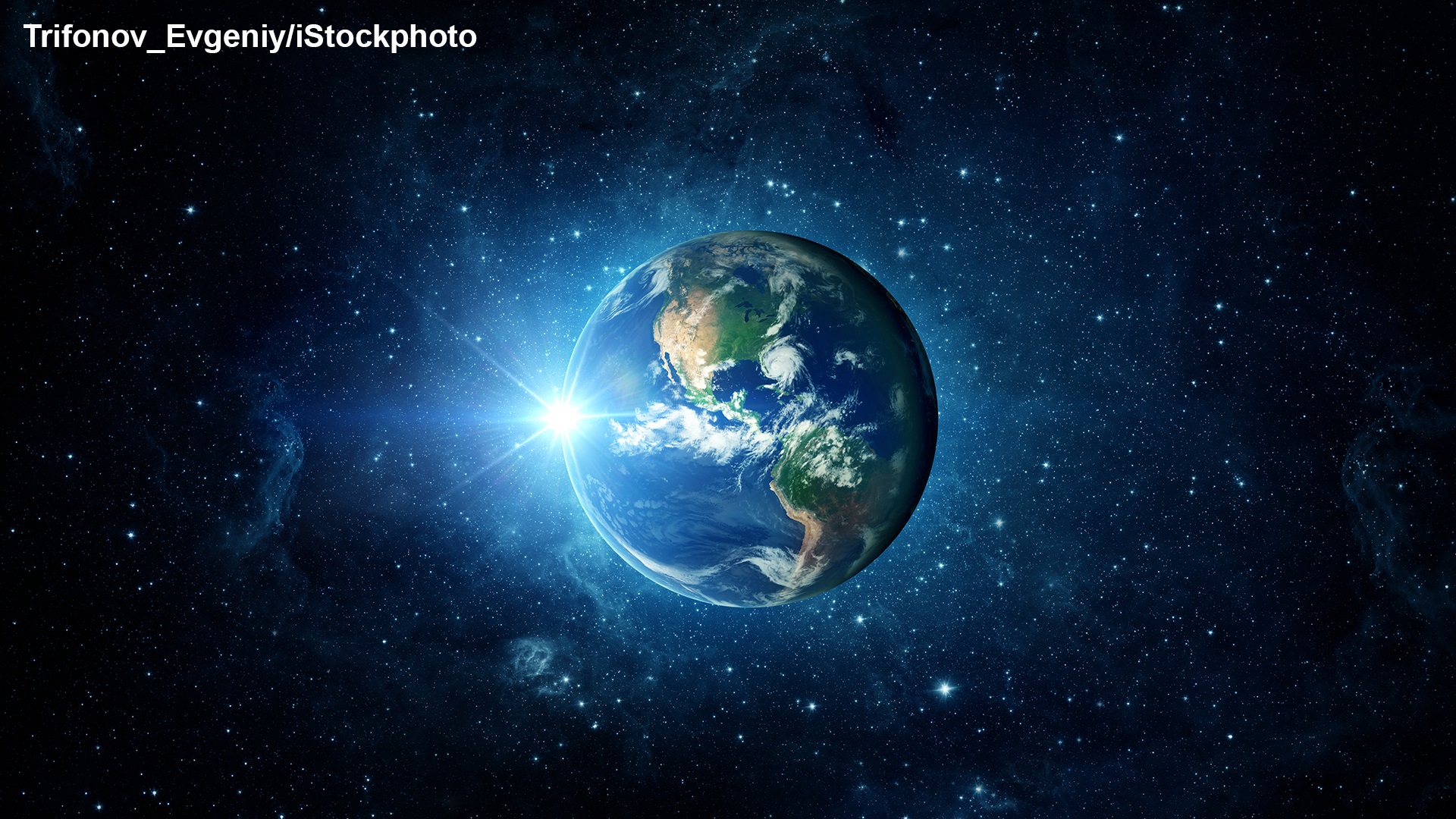
by Hanna Kurlanda-Witek
The story of how life formed on Earth keeps unfolding, revealing new clues about how our planet evolved from being barren and hostile to life, wrapped in harmful gases, to the lush and diverse place we know today. The problem associated with the formation of the first cellular organisms – or the organic compounds from which they are built – is that these processes took place over four billion years ago and much of the evidence has ceased to exist. As Nobel Laureate George Smoot mentioned during his lecture in Lindau in 2008, this area of research is not unlike forensic science, where evidence is collected just like at a crime scene. Author Peter Forbes wrote that the origin of life “could once be safely consigned to wistful armchair musing – we’ll never know (...)”. Nevertheless, the topic has not ceased to fascinate scores of scientists, among them Nobel Laureates. Even though Nobel Prizes are not given for abiogenesis or evolutionary biology, many laureates have shifted their research interests to this exciting field after winning the Nobel Prize in another discipline. Harold Urey, who won the Nobel Prize in Chemistry in 1934 “for the discovery of heavy hydrogen”, dedicated many years to the early geochemistry of planets and meteorites; his lectures at the Lindau Meetings in the 1960s and 1970s focused on the chemical evidence of the origin of the solar system. Christian de Duve is probably equally famous for his discovery of the cellular organelles lysosomes and peroxisomes, for which he received the Nobel Prize in Physiology or Medicine in 1974, as for his many books on the rise of life.
Recent developments in molecular biology, genomics and biochemistry have added even more clues to theories of abiogenesis. De Duve wrote in 1995: “The history of life on earth is written in the cells and molecules of existing organisms. Thanks to the advances of cell biology, biochemistry and molecular biology, scientists are becoming increasingly adept at reading the text.” There is much ongoing debate as to how probable the formation of life was. If we went back in time, would the same processes occur? Or would Earth be colonised by decidedly different organisms (or none at all)? The origin of life was a singular occurrence that led to a sequence of events – one offshoot coming from one type of model formation, others leading to evolutionary dead ends. During the 1994 Lindau Nobel Laureate Meeting dedicated to Physics, the physicist Murray Gell-Mann presented a theory that could be echoed by evolutionary biologists as well as chemists, which stated that complexity arises from simplicity, or from an initial order, and is then subject to chance.
(00:01:46 - 00:02:54)
The Big Bang occurred 13.7 billion years ago, producing the first elements – hydrogen and helium. As John Mather explained in his lecture in 2010, a small piece of the primordial material started to expand very quickly, at a rate that was faster than the speed of light. Yet, our local universe is not expanding and this can be explained by the inhomogeneity of some parts of the early universe; some were denser than others, which led to an uneven distribution of matter, allowing for the formation of the solar system approximately 4.5 billion years ago. Nuclear reactions taking place inside stars initiated the formation of chemical elements, hence all matter today is the recycled material left over from those early stars.
(00:10:44 - 00:12:26)
Life took its time to produce complicated organisms such as ourselves, but once the process began it never stopped. The Earth was only physically able to bear life approximately four billion years ago, half a billion years after the solar system was born. Half a billion years after the Earth possessed water and adequate temperatures, the first prokaryotes, or unicellular organisms without nuclei, appeared on the scene. Earth was home to only these very small and simple organisms for over 1.5 billion years, eventually giving rise to blue-green algae, or cyanobacteria, which used energy from the sun to photosynthesise, releasing oxygen as a by-product in the process. Two billion years ago marked the rise of eukaryotes, single cell organisms with nuclei; yet, another one billion years were to pass before multicellular organisms came to life. The development of complex organisms speeded up; the first marine vertebrates appeared 600 million years ago, and the first primates only 60 million years ago. Although we are not able to see this timeline, in 2010, Christian de Duve gave a brief description of the chronology of events in Earth’s biological history:
(00:02:00 - 00:07:56)
As de Duve mentioned here, this timeline has only been known to us for the last several decades. The questions we ask today go back even further, to the very beginnings of the timeline. How were the first proteins and nucleic acids formed and did this occur simultaneously? What were the origins of primitive cellular life? Scientists are now trying to experimentally decipher “the chemistry of a young planet to the beginnings of biology” in the words of Nobel Laureate Jack Szostak. One of the first experiments in abiotic chemistry took place in 1953, when Nobel Laureate Harold Urey’s graduate student, Stanley Miller, sought to examine what effect lightning may have had on the Earth’s early atmosphere. Two flasks were linked together, one containing water, the other one a mixture of methane, hydrogen, ammonia and water vapour, and these were subjected to electrical discharges imitating lightning. After a few days, it was found that many organic compounds had developed in the flasks, among them amino acids. This now-famous experiment, known as the Urey-Miller experiment, generated a lot of attention but has since become invalid as scientists now believe the early atmosphere contained mostly nitrogen and carbon dioxide, which would not be as conducive to life. Also, the experiment lacked an example of redox chemistry, or the transfer of electrons from donors to acceptors in order to produce carbons.
For many years, the major dilemma centred on the unlikely synchronous establishment of proteins and DNA. “Proteins can’t exist without DNA and DNA has no purpose without proteins,” wrote Bill Bryson in his book “A Short History of Nearly Everything”. As Jack Szostak explained in his lecture at the Lindau Meeting in 2015, starting from the 1960s, molecular biologists theorised that early life could be much simpler; perhaps there was a molecule that could act as an enzyme and could catalyse its own replication – the all-in-one biological macromolecule. Experiments conducted by Sidney Altman and Thomas Cech in the 1970s demonstrated that RNA possessed these catalytic properties, a discovery for which the two were awarded the Nobel Prize in Chemistry in 1989. Here, Szostak introduces us to the “RNA world”:
(00:04:07 - 00:08:04)
Ribosomes are cell structures responsible for deciphering RNA and forming polypeptides out of amino acids. Research conducted by Nobel Laureate Ada Yonath’s group has suggested the structure of the ancestral ribosome – the proto-ribosome, in which the prebiotic bonding is still abundantly functioning in our own bodies. During the 66th Lindau Nobel Laureate Meeting, Yonath explained the dimer hypothesis, stating that the proto-ribosome formed from two foldable RNA chains. The hypothesis also states that only those constructs that had a tendency to dimerise in this fashion could evolve, leading to the conclusion that even molecules were susceptible to Darwinian selection.
(00:21:14 - 00:24:55)
But molecules themselves aren’t enough to produce life – a cellular membrane is required in order for the protometabolism to function and replication to occur, as it is the proximity of the molecular components encapsulated within a protective membrane that enable the simple cellular structure to work. Szostak described the schematic “protocell” with membranes made out of fatty acids and fatty alcohols, which are more permeable and dynamic than our own phospholipid cell membranes:
(00:10:08 - 00:13:25)
For the primitive cell to function, it requires a membrane, a means of replicating genetic information and a source of external energy to operate the cell machinery. The surrounding environment of these primitive cells must be favourable for these conditions to occur. During his lecture dedicated to microbes, Richard Roberts described hydrothermal vents in the ocean floor, also known as black smokers. These chimney-like structures made from iron sulphide were discovered near the Galápagos Islands in 1977, and are the result of magma mixing with seawater. Many different organisms live close to these hydrothermal vents, and many theories link these environments to early life:
(00:09:10 - 00:11:32)
Jack Szostak further explained what environmental conditions were necessary:
(00:29:32 - 00:31:03)
In 1989, William Martin from the University of Düsseldorf and Michael Russell of NASA’s Jet Propulsion Laboratory pointed out that black smokers were too acidic and the temperatures too great to support the growth and proliferation of primitive life. They suggested that it is more probable that life originated in alkaline hydrothermal vents, a hypothesis known as the “water world” theory. These vents are cooler than black smokers, and the alkaline fluids bubbling from the vents could have been more amicable to the first life-forms than the surrounding acidic oceans. At this boundary, two chemical imbalances could take place: a proton gradient and an electrical gradient, which led to electron transfer. Both of these gradients occur in mitochondria, cell structures which produce ATP (adenosine triphosphate), an energy-carrying molecule. Alkaline hydrothermal vents are the main source of hydrogen on Earth, and when hydrogen mixed with carbon dioxide, which was abundant in early oceans, organic compounds, such as thioesters, could have formed. De Duve viewed this event as “primeval in the development of life”. Amino acids, present in the form of thioesters, could spontaneously assemble into peptides in bacteria, as demonstrated by Nobel Laureate Fritz Lipmann in the late 1960s. Moreover, the minerals found in these vents, such as iron-sulphur clusters, may have brought about iron-sulphur compounds. Evidence of this is present in the enzymes of many organisms, a sort of remnant passed down from unicellular ancestors.
The pathways of survival of the fittest began with the earliest life-forms, even at a molecular level, as was mentioned in Ada Yonath’s lecture. De Duve stated in Lindau in 2011 that variation brings about competition and that natural selection operates on the here and now. In his lecture in 1990, Werner Arber explained that evolution is not aimed at any particular group, it is a completely random occurrence and is dependent on changes in living conditions and the surrounding environment. In light of experiments in biological evolution, it is easier to track mutations in microorganisms.
(00:14:13 - 00:15:45)
(00:02:20 - 00:06:24)
Ian Tattersall called humans “a very egotistical species”. Many of us feel that evolution progressed in a linear fashion, culminating in ourselves, whereas no such evidence exists, unless to support the current theory that many evolutionary sidelines developed and eventually disappeared. For billions of years, Earth was home to life in the form of single cells, and, as Nobel Laureate Richard Roberts explained in Lindau in 2007, most of the diversity and mass on Earth are still accounted for by microbes, which we often underestimate simply because they are too small to see:
(00:03:16 - 00:05:51)
Roberts finished his talk by proposing, “Perhaps humans were invented by bacteria in order to provide a nice place to live.”
The transition period between prokaryotes and eukaryotes is a critical chapter in Earth’s early evolution. The defining moment took place when oceanic cyanobacteria started to produce oxygen, which, approximately 300 million years later, led to the so-called Great Oxygenation Event 2.4 billion years ago. However, the earliest fossil records demonstrate that eukaryotes appeared 1.4 billion years ago. It took about a billion years, but the accumulation of oxygen in the Earth’s atmosphere finally gave aerobic and more complex organisms the upper hand.
(00:05:53 - 00:06:57)
There are many models of how the nucleus and mitochondria, important for aerobically respiring organisms, came into being. These models originated from the endosymbiotic theory propagated by Lynn Margulis in 1967. A host bacterium collected another bacterium and with time the “swallowed” bacterium became part of the host cell. Fritz Lipmann described the process during his lecture in 1978, at a time when the endosymbiotic theory was not as universally accepted as it is today:
Bill Bryson succinctly summarised eukaryotic cells’ relationship with mitochondria: “(...) yet even after a billion years mitochondria behave as if they think things might not work out between us. They maintain their own DNA, RNA and ribosomes. They reproduce at a different time from their host cells. They look like bacteria, divide like bacteria and sometimes respond to antibiotics in the way bacteria do. They don’t even speak the same genetic language as the cell in which they live. In short, they keep their bags packed. It is like having a stranger in your house, but one who has been there for a billion years.”
The plastids, such as chloroplasts – organelles in photosynthesising eukaryotes – are believed to have evolved from cyanobacteria, also as a result of endosymbiosis.
There are a great many questions still to be answered regarding life’s origins, laying the foundations for debate as theories and counter-theories, which are based on the findings of biochemists and molecular biologists but also geologists and geochemists, flourish. Yet, what is truly captivating is that these labyrinthine developments have eventually brought forth humans, who are increasingly able to solve puzzles of life’s origins across the expanse of four billion years and more.
Further Readings:
https://www.jpl.nasa.gov/news/news.php?feature=4109
http://oceanservice.noaa.gov/facts/vents.html
Bryson, B. (2003). Chapter 19: The Rise of Life, in: A Short History of Nearly Everything. Doubleday.
De Duve, C. (1995). The Beginnings of Life on Earth. American Scientist, September-October issue.
Forbes, P. The Vital Question by Nick Lane – a game-changing book about the origins of life. The Guardian, Wednesday, 22 April, 2015.
Lane, N. (2010). Chance or Necessity? Bioenergetics and the Probability of Life. Journal of Cosmology 10, pp. 3286-3304.
Martin, W.F., Garg, S. and Zimorski V. (2015). Endosymbiotic theories for eukaryote origin. Philiosophical Transactions B. 370.