Prof. Dr. John Bardeen > Research Profile
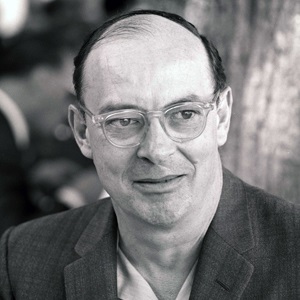
By Roberto Lalli
John Bardeen
Nobel Prize in Physics 1956 together with William B. Shockley and Walter H. Brattain
"for their researches on semiconductors and their discovery of the transistor effect".
John Bardeen occupies a unique place in the pantheon of Nobel Prize Laureates. Up to this day, he is the only scientist to have won two Nobel Awards in the same category: physics. The successes Bardeen achieved were in large part related to the circumstance that his research enterprises were situated at the boundary between industrial and academic environments, which Bardeen crossed more than once during his life. Believing that there was no fundamental distinction between pure and applied science, Bardeen was a theoretical physicist who since the beginning of his career focused on the application of quantum mechanics to solid-state physics. In this field, Bardeen made several major contributions, but he is especially remembered for the achievements that gained him the two Nobel Prizes in physics. The first was the discovery of the point-contact transistor in 1947, along with his co-worker Walter Brattain at the AT&T Bell Telephone Laboratories (Bell Labs in short). After the discovery of the transistor had dramatically transformed the field of electronic communications, the two Bell Labs physicists shared the 1956 Nobel Prize in Physics with their colleague William Shockley who invented the junction transistor in 1948. Worsening of the working conditions led Bardeen to leave Bell Labs and accept an academic position at the University of Illinois at Urbana-Champaign in 1951. There, he conducted research aimed at understanding the phenomenon of superconductivity. In 1957, along with the postdoctoral associate Leon Cooper and his PhD student John Schrieffer, Bardeen put forward the first successful microscopic theory of superconductivity. The Bardeen-Cooper-Schrieffer theory, better known as BCS theory, was able to make precise predictions about many empirical phenomena concerning superconductivity as well as the phase transition between the normal and the superconducting states. After a number of physicists contributed to clarify its deep implications, the BCS theory became the orthodox microscopic description of superconductivity. In 1972, fifteen years after the publication of their breakthrough paper, Bardeen received the second Nobel Award in Physics, along with his co-workers Cooper and Schrieffer.
Looking for a non-academic career path
John Bardeen was born in Madison¬—the capital of Wisconsin—on the 23rd of May 1908, to the Professor of Anatomy and Dean of the Medical School of the University of Wisconsin at Madison Charles R. Bardeen and his wife Althea Harmer. Since an early age, Bardeen showed great inclination for natural sciences graduating from Madison Central High School at the remarkably young age of fifteen. Confronted with the decision about his undergraduate studies, Bardeen chose electrical engineering, the main rationale being that he did not want to follow his father’s steps and become an academic. Electrical engineering, in his view, offered the opportunity to follow his natural inclination towards natural sciences, while, at the same time, allowing him to pursue a rewarding career in industry. In 1923, Bardeen enrolled at the University of Wisconsin at Madison and, five years later, he earned his bachelor after having gained some experience at the engineering laboratory of the Western Electric Company in Chicago.
After graduation, Bardeen continued as a graduate student at the same university, mainly working on mathematical problems in applied geophysics. In this period, Bardeen was introduced to quantum mechanics by John H. van Vleck—at the time one of the few American theoretical physicists working on the recently developed quantum mechanics and an expert of the application of the theory to the solid state. Van Vleck would receive the Nobel Prize in Physics, along with Philip W. Anderson and Nevill F. Mott, “for their fundamental theoretical investigations of the electronic structure of magnetic and disordered systems” in 1977.
Bardeen earned his M.Sc. with a thesis on electrical prospecting for oil under the supervision of the American geophysicist Leo J. Peters in 1929. Unfortunately, the 1929 stock market crisis had rapidly deteriorated the job prospect for a young and brilliant electrical engineer as Bardeen undoubtedly was. Thus, Bardeen decided to remain at the university making research on theoretical problems concerning antennas. After having evaluated the very few job offers, Bardeen eventually followed his thesis advisor to the Gulf Research Laboratories in Pittsburgh, Pennsylvania. There, Bardeen worked the next three years on the development of methods for the interpretation of magnetic and gravitational surveys.
Return to pure science
The experience at the Gulf Research Laboratories was not completely satisfying, and Bardeen resolved to enrol at Princeton University to pursue PhD studies in mathematical physics in 1933. In Princeton, Bardeen became interested in solid-state physics and in the application of quantum mechanical laws to many-body systems. His supervisor was the Hungarian Jewish physicist Eugene Wigner, who was working at Princeton University since 1930 and had recently decided to stay in the United States because of the growth of anti-Semitism and the worsening of the political climate in Germany after the rise to power of the Nazis.
Wigner had been working on the development of the theory of groups and its application to quantum mechanics since 1927. Recently, Wigner had got interested in the application of quantum mechanics to the theory of solids and Bardeen made his PhD research on such a topic. Along with other two PhD students of Wigner’s—Frederick Seitz and Conyers Herring—Bardeen was part of the first generation of American physicists trained to apply quantum mechanics to real solids together with the group of John Slater’s graduate students at MIT. Building on the work Wigner was carrying on with Seitz on the quantum mechanical computation of the approximate band structures of sodium, Bardeen developed a method for calculating the work function of metals; i.e., the minimum energy necessary to remove an electron from the surface of a metal to infinity.
Even before receiving his PhD, Bardeen became Junior Fellow in the eminent Harvard’s Society of Fellows. At Harvard, Bardeen remained three years from 1935 to 1938 pursuing research on the application of quantum mechanics to many-body problems. At that time, one of the most important targets was the development of theoretical tools that allowed the extension of quantum mechanical laws to large aggregates of simple entities. Bardeen struggled to work out meaningful expressions for the description of the interactions between electrons as well as between electrons and the ionic lattice with the hope to develop a reliable theory of superconductivity—the phase transition of certain metals and alloys that completely lose their electrical resistance below a certain temperature, called critical temperature. However, all his attempts met with frustration because of the lack of adequate theoretical techniques.
Nevertheless, his Harvard experience was very fruitful, especially because he worked in close contact with two eminent physicists who had a long-lasting influence on Bardeen’s future works: Van Vleck and Percy Bridgman. Van Vleck continued to be a guiding light for what concerned the most novel advancements of quantum theory. Bridgman, on the other hand, was an American experimental physicist who had already been appreciated as the World’s leading expert on high-pressure physics, for which he would be awarded the Nobel Prize in 1946. Bardeen worked together with Bridgman in order to provide theoretical models for the experimental data that Bridgman was recording. This kind of close connection with the experimenter’s work would rapidly become a key feature of Bardeen’s style of doing research, which would play a fundamental role in the discovery of the point-contact transistor.
While at Harvard, he became acquainted with Shockley who was also working on the application of quantum mechanics to materials, after having pursued a doctoral research entitled Electronic Bands in Sodium Chloride under Slater at MIT. In 1938, Shockley accepted a research position at Bell Labs where he was later invited to build a research group devoted to the study of the properties of semiconductors. Shockley was instrumental in bringing Bardeen to Bell Labs after the Second World War, when Bardeen was called to become a member of the newly born group.
Research at Bell Labs and the invention of the transistor
After a two-year period spent at the University of Minnesota and another three years devoted to war-related research in electrical engineering at the Naval Ordnance Laboratory during World War II, Bardeen joined the semiconductor group headed by Shockley at Bell Labs in late 1945.
Since its establishment in 1925, Bell Labs had been strongly committed to pure research. One of the convictions of Bell Labs’ first president—Frank B. Jewett—was that research not strictly related to short-term needs of the firm should be pursued because it would provide new technologies that might become fundamental in the long run. In the early 1930s, the newly appointed director of research of Bell Labs—Mervin J. Kelly—began creating a group of theoretical physicists who would explore novel theoretical advancements that might possibly be useful for communication technology. One of the most important targets was to find a better alternative to vacuum tubes as amplifier for telephone lines. Since the beginning of the 20th century, vacuum tubes had been successful in providing a reasonably economic means for the amplification of electrical signals. Various technological improvements had made these devices more and more efficient, but they still had several shortcomings—the most important of which were their fragility, their large dimension and the fact that they consumed a lot of power.
Shockley had begun investigating solid-state amplifiers in 1938. After the end of the war, he was invited to build a small research group whose target was to explore semiconductors as possible amplifiers, an alternative to vacuum tubes. Bardeen was one of the members of the small group along with the experimental physicist W. Brattain, G.L. Pearson (who was mainly concerned with bulk properties), the physical chemist Robert Gibney, and the circuit expert Helbert Moore. None of them had worked on semiconductors during the war. However, the group could make good use of the several improvements made in war-related research activities. In particular, since semiconductors had been employed in radar detectors, several techniques had been developed to obtain highly purified germanium and silicon. Because of the development of these novel techniques, germanium and silicon were considered the ideal elements for conducting research on semiconductor amplifiers. It was, indeed, relatively easy to control the electrical properties of these materials by introducing appropriate amounts of donor and acceptor impurities.
Soon after his hiring, Bardeen focused on the development of the theory of semiconductors building on the advancements made before the war; especially, Alan Wilson’s quantum mechanical theory of the energy band model, Victor Frenkel’s theory of photoconductive phenomena, and Walter Schottky’s mathematical theory of contact rectification—the modification of current from direct to alternate.
Although the idea that semiconductors could be used as amplifiers seemed reasonable, there were strong uncertainties about how to build this kind of device. In 1945, Shockley suggested to apply a strong transverse electric field to a slab of semiconductor material in order to modify its conductance—an effect that came to be called field effect. According to Shockley, a strong transverse electric field would have produced a significant amplification in an alternating current signal. Various experimental tests of Shockley’s hypothesis were performed at the Bell Labs, which showed negligible or null amplification. Shortly afterwards, Bardeen was asked to investigate why Shockley’s theory did not work as expected.
This series of events led Bardeen to investigate the theory of surfaces—a topic he had already encountered during his PhD research. Bardeen suggested that a large part of the surface electrons in semi-conductors were immobilized in specific states and that, for this reason, they could not participate to conduction. After intensive experimental research conducted at Bell Labs and elsewhere showed that there was enough evidence for the existence of free surface states in semi-conductors, Bardeen began working with Brattain to modify the levels of surface barriers. The employment of an electrolyte seemed a possible method to overcome the surface states and construct an amplifier. Instead of working with a thin film of semiconductor material obtained by evaporation, Bardeen and Brattain used a slab of p-type silicon and produced an inversion layer of n-type conductivity by oxidising the surface. After further modifications of the apparatus, Bardeen and Brattain produced a device that actually amplified electric signals in a very effective way, but different from the field effect on which they were working. The apparatus they created allowed holes (empty electron states that acted as positive charges) to flow into the block of n-type germanium. The final design, suggested by Bardeen, was based on the linking of the germanium to two slightly separated metal point contacts made of gold. In this way, the holes introduced into the germanium through the positively charged point contact (the emitter) could flow rapidly away through the negatively charged point-contact (the collector). The new device was tested on December 16, 1947 and it showed considerable voltage, current, and power amplification. The Bardeen-Brattain device was the first solid-state electronic transistor ever created—an invention that would rapidly change the field of electronics.
Shockley—who was not directly involved in the discovery of the point-contact transistor—made many efforts to produce a new device, at the same time preventing his collaborators Bardeen and Brattain from further improving the newly discovered transistor. In a few months, Shockley put forward a second type of transistor called junction device, to the development of which he had worked secretly, while Bardeen and Brattain were busy in drafting patent applications. In June 1948, to the surprise of his collaborators, Shockley announced the discovery of the junction transistor, which would rapidly supersede the Bardeen-Brattain point-contact transistor for the majority of commercial applications of solid-state amplifiers. The discovery of the transistor was soon considered one of the major technological advancements of the 20th century, and transistors became ubiquitous in the matter of a few years. For this momentous discovery, Bardeen, along with Brattain and Shockley, was awarded the Nobel Prize in Physics in December 1956.
The BCS theory of superconductivity
Notwithstanding the enormous success of the transistor, the working environment at Bell Labs rapidly deteriorated because of Shockley’s behaviour following Brattain and Bardeen’s invention of the point-contact transistor. Bardeen decided to dedicate himself to the theoretical understanding of superconductivity, which was one of the most important open problems of solid-state physics.
The Dutch physicist Heike Kamerlingh Onnes—one of the pioneers of low-temperature physics—discovered the phenomenon of superconductivity in his laboratory in Leiden on April 8, 1911. Kamerlingh Onnes’ collaborator Gilles Holst observed that the resistance of a solid mercury wire went abruptly to zero at 4.2 K. In his 1913 Nobel lecture, Kamerlingh Onnes emphasised the importance of the discovery maintaining that mercury had “entered a new state,” which he christened superconductivity. Further experiments showed that various metals and alloys became superconductors once they were cooled below the critical temperature, which depended on the material. In 1913, another important property of superconducting states was discovered: Superconductivity disappeared once the material was immersed in a sufficiently strong magnetic field.
After the development of quantum mechanics, many of the pioneers of the novel theoretical framework, along with their students and younger associates, saw the properties of solids as a natural field for the application of the quantum theory to complex systems, in which the interactions between many particles played a major role. Between 1928 and 1933 important progresses in the quantum theoretical understanding of solid-state physics were made. A. Sommerfeld, W. Heisenberg, W. Pauli, along with their PhD students and research associates, put forward many of the building blocks of the theory of solids on the basis of the laws of quantum mechanics and their applications to the interactions between the ionic lattice and the electrons. Since 1929, superconductivity was considered a fundamental part of the research programme aiming at the quantum theory of solids, and there was much hope that a reliable microscopic theory of superconducting states would shortly emerge. Between 1929 and 1933, more than a dozen theoretical physicists, including founding fathers of quantum mechanics such as N. Bohr, Pauli and Heisenberg and brilliant younger experts of the application of the new theory to the properties of solids, like F. Bloch and L. Landau, tried to put forward a microscopic theory of superconductivity. But all these attempts failed. In 1933, superconductivity still maintained the status of an unsolved problem, while many other many-body phenomena had been successfully dealt with in the quantum mechanical framework.
In 1933, the German physicists W. Meissner and R. Ochsenfeld discovered that a material in the superconducting state was a perfect diamagnet. It was soon recognized that the Meissner effect—as the expulsion of weak magnetic fields from a superconductor when it is cooled below the critical temperature was shortly baptised—was a fundamental feature of the phase transition between normal and superconducting states. To many, perfect diamagnetism seemed the best way to characterize the superconducting states rather than the vanishing resistance. The discovery of the Meissner effect, indeed, led to the development of the first successful phenomenological theory of superconductivity put forward by the German physicists Fritz and Heinz London in 1935, which, a posteriori, was regarded as a major step towards the theoretical understanding of the phenomenon. Of Jewish family, the London brothers had recently fled from Nazi Germany and begun working at the Clarendon Laboratory in Oxford, where they collaborated with other refugees in the establishment of the first Oxford low-temperature physics programme.
The London theory was based on a two-fluid model—according to which electrons were separated in normal and superconducting, and the proportion between them depended on the temperature—and an equation relating the superconducting current density and the vector electromagnetic potential A within the superconductor, for an appropriate choice of gauge (the Coulomb gauge divA=0). The combination of London and Maxwell equations explained various properties of superconductors including the vanishing resistance and the exponential decay of the magnetic field inside the superconductor with a characteristic penetration depth. In the diamagnetic picture provided by the London theory, supercurrents were determined by the magnetic fields and were metastable phenomena; i.e., configurations in which the system spent an extended time, but that were different from the state of least energy of the system. To provide some microscopic grounds to their phenomenological theory, the London brothers hypothesised that the ground-state wave function of a superconductor remained independent (“rigid” as they defined it), at least to a first approximation, from an impressed weak magnetic field.
Although the London theory provided a phenomenological account of some of the most relevant properties of superconducting states, it was considered largely unsatisfying for several reasons. From the experimental perspective, the London theory did not account for the different empirical properties of the various metals and alloys that showed the property of superconductivity. On the theoretical side, the necessity was strongly felt to find a microscopic theory of superconductivity based on quantum mechanical laws.
Significant theoretical advancements occurred only after World War II. In 1950, building on his theory of general second order phase transitions, the Soviet theoretical physicist Lev Landau along with Vitaly L. Ginzburg proposed a theory of superconductivity based on the power series expansion of the free energy difference between normal and conducting states in a complex order parameter ω, which was defined so that ω is 0 in the normal state, while ω=1 in the superconducting state at T=0°K. The order parameter was identified as the square of an effective wave function ψ, defined so that the absolute value of ψ2 was equal to the density of superconducting electrons. By minimizing the free energy, Ginzburg and Landau derived a series of equations relating the order parameter, the supercurrent and the vector potential. The Ginzburg-Landau equations allowed for the treatment of the boundary between normal and superconducting regions. Moreover, it was the first theoretical approach to suggest that there were two different kinds of superconductors, with different reactions with respect to the applied magnetic fields. As was later recognized, type-I superconductors were those superconductors in which the superconducting phase is abruptly destroyed as soon as the applied magnetic field reaches a certain critical value. In type-II superconductors, instead, there is an intermediate phase characterised by the formation of magnetic vortices when the magnitude of the applied magnetic field lies between two different critical values H1 and H2, while the material comes back to the normal state for values of the magnetic field greater than H2.
On the experimental side, several groups made important progress in the clarification of various aspects of the phenomenon, including the correlation between penetration depth and amount of impurities, and the relations between the transition temperature and the isotopic mass of atoms in the lattice. In 1950, two experiments carried out in the United States to explore the latter point by measurements on samples of different mercury isotopes showed a surprising result. There was a proportional relation between the critical temperature and the mass of the isotopes: The value of the critical temperature varies inversely with the square root of the mass of the atomic lattice. This isotope effect—as the experimental finding was shortly named—suggested that the phenomenon of superconductivity depended on the coupling between conduction electrons and the lattice vibrations.
This latter result was instrumental in revitalising Bardeen’s interest in superconductivity. Unsatisfied with the working environment at Bell Labs, where there was scant interest in supporting theoretical research on superconductivity, Bardeen decided to pursue an academic career, accepting a professorship at the University of Illinois at Urbana-Champaign in 1951. There, he carried on the project to develop the microscopic theory of superconductivity with various collaborators. Bardeen began exploring the techniques developed by D. Bohm and D. Pines to treat the electron-electron interactions in plasmas. From 1951 to 1953, Bohm and his graduate student Pines had published a series of important papers on the collective behaviour of electron-electron interactions providing the building blocks for the theoretical description of the quantum electron gas. Bardeen was successful in securing Pines as his postdoctoral assistant at the University of Illinois. With Pines, Bardeen worked on the extension of the Bohm-Pines theoretical framework to the description of superconducting states by taking into account the ionic motion. After having developed their formalism for the phonon-electron interaction, Bardeen and Pines showed that, for interactions in which the energy transfer was small, the coupling of lattice vibrations and electrons resulted in an attractive force between electrons that overcomes the screened Coulomb repulsion, suggesting in this way a microscopic mechanism for superconductivity.
In 1953, Bardeen was asked to write a review on the theoretical research on superconductivity for the recently established series Handbuch der Physik edited by S. Flügge. At that time, Bardeen was already convinced that the properties of superconducting states might be derived by means of a reasonable energy-gap model, a model that implies an energy gap between the superconducting ground state and the single electron first excited state. Bardeen’s work on the long review allowed him to deeply study all the available scientific literature on the theory of superconductivity. In his 96-page long article Bardeen stated that it was necessary to put forward “radically new ideas” to get a good physical picture of the superconducting states. Bardeen was determined in developing the new theory and the needed novel concepts with his group. In particular, he was recognizing that field theoretical techniques could be the right theoretical tools for the description of the quantum Fermi gas in superconductors and its interconnection with the ionic lattice. The new quantum electrodynamics developed by the late 1940s was a model on which one could perhaps rely in order to describe superconductivity in quantum field theoretical fashion. To achieve this target, Bardeen brought to Urbana as a postdoctoral associate Leon Cooper, who had recently completed his PhD on theoretical nuclear physics. Cooper joined the Bardeen group soon after Pines had left the University of Illinois in 1955 to accept a teaching position at Princeton.
The third member of the small group working on superconductivity was J. Robert Schrieffer—a graduate student who was pursuing his PhD under Bardeen’s supervision.
After the group joined their talents, they were able to make quick progress in the matter of months. The first important step towards the resolution of the problem was made by Cooper in 1956. Cooper demonstrated that if the net force between two electrons lying outside the Fermi surface is attractive, then the two electrons form a bound state separated by an energy gap from the continuum state however weak the attractive force might be. The Cooper pairs—as Copper’s result was shortly christened—were considered as a possible essential ingredient of the microscopic theory of superconductivity. It was, however, still necessary to find a many-body wave function that took into account the large number of overlapping electron pairs.
While Bardeen was travelling in Europe to receive his Nobel Prize for the discovery of the transistor he gave his co-workers the task to find the ground state wave function of superconductors with Cooper pairs. In January 1957, Schrieffer solved this problem while riding on a subway in New York City. He wrote down a many-body wave function as a product of creation operators acting on vacuum, which gave terms with changing total number of Cooper pairs. The BCS theory of conductivity was born. After Schrieffer announced his result when Bardeen came back from Sweden, the three physicists began applying the new theory to a series of specific cases to check if the theoretical predictions were confirmed by the empirical data. Bardeen decided to publish a short letter with the outline of the theory in Physical Review in order to secure priority for their discovery, even though they had not been able to derive the second-order phase transition between the normal and superconducting states at the critical temperature. Bardeen was invited to present the theory to the March 1957 meeting of the American Physical Society, but he decided to send Cooper and Schrieffer, because he did not want to overshadow his collaborators and wished that the young people got the right credit. (Eventually, the two talks were presented by Cooper alone, because Schrieffer could not arrive in time). After further progress had been made and some experimental confirmation had increased the confidence of the authors in their theory, Bardeen, Cooper and Schrieffer published the 30-page version of their discovery in the paper “Theory of Superconductivity,” published in Physical Review on December 1, 1957. By the time the paper was printed, the essential elements of the theory were already known, and several debates on its validity had taken place in various settings. Notwithstanding its immediate success, the theory was not well received by other theoreticians. Some of the terms in the theory seemed unsound from a physical perspective, and it required some years before the physical implications of the theory were fully understood, especially in terms of broken symmetry—a perspective that was not explicitly present in the original papers by Bardeen, Cooper and Schrieffer.
After the theory had finally gained consensus within the physics community and the BCS theory had also become a model for the application of symmetry breaking to particle physics, Bardeen, Cooper and Schrieffer were awarded the Nobel Prize in Physics in 1972, “for their jointly developed theory of superconductivity, usually called the BCS-theory.”
A career between academia and industry
After having left the Bell Labs for a professorship at the University of Illinois, Bardeen did not completely abandon the world of industry. This was perfectly coherent with Bardeen’s general view that the most meaningful scientific researches were those that promised of having practical applications. He continued to maintain a series of working connections with industrial firms, especially with the Haloid Company of which he was a scientific consultant from 1952 onward. In 1961—the year in which Haloid was renamed Xerox Corporation—Bardeen became a member of its board of directors. He eventually stepped down from all his roles within the firm in 1980 after almost thirty years of strong commitment to the technological innovations and long-terms goals of the company.
When he was already recognized as one of the most outstanding American physicists working on theoretical physics devoted to useful practical applications, Bardeen was asked to serve in national advisory panels. Among other duties, from 1959 to 1962, he served as a member of the President science Advisory Committee of D. Eisenhower and J. F. Kennedy. Notwithstanding his hesitancy, Bardeen was also convinced to sit in President Reagan’s White House Science Council from 1981 onward, but he rapidly resigned after Reagan began the missile program called Strategic Defence Initiative (SDI) without consulting the Council.
On the scientific side, after the development of the BCS theory and its acceptance as the most valuable microscopic theory of superconductivity, Bardeen became one of the most influential authorities in solid-state physics and his opinions had a major impact in various debates about further discoveries about many-body problems. During the 1980s, he became primarily interested in the development of a new quantum mechanical theory of charge density waves (CDWs)—a periodic modulation of the electronic charge density. According to Bardeen, the CDWs might be explained as a macroscopic quantum phenomenon on the same ground as superconductivity. While initially interested in Bardeen’s theory, the condensed matter community eventually came to prefer more classical approach to the CDWs, which led to a predictable relationship between the level of impurities and the threshold voltage above which the sliding of the CDWs occurs. This evaluation of his theory led Bardeen to feel increasingly isolated in the community he had contributed to shape. He continued to publish papers on the theoretical description of CDWs and on the newly discovered phenomenon of high-temperature superconductivity till January 1991, when he died of massive heart attack at the age of 82.
Bibliography
Bardeen, J (1956) Theory of Superconductivity. Theoretical Part, Handbuch der Physik 15: 274-369.
Bardeen, J. (1956) Nobel Lecture: Semiconductor Research Leading to the Point Contact Transistor. Nobelprize.org. Nobel Media AB 2014. Retrieved 10 December 2014. http://www.nobelprize.org/nobel_prizes/physics/laureates/1956/bardeen-lecture.html
Bardeen J. (1972) Nobel Lecture: Microscopic Quantum Interference Effects in the Theory of Superconductivity. Nobelprize.org. Nobel Media AB 2014. Retrieved 10 December 2014. http://www.nobelprize.org/nobel_prizes/physics/laureates/1972/bardeen-lecture.html
John Bardeen - Biographical. Nobelprize.org. Nobel Media AB 2014. Retrieved 16 December 2014. http://www.nobelprize.org/nobel_prizes/physics/laureates/1972/bardeen-bio.html
Hoddeson, L. (1981) The Discovery of the Point-Contact Transistor. Historical Studies in the Physical Sciences 12: 41–76.
Hoddeson L. (1981) The Emergence of Basic Research in the Bell Telephone System, 1875–1915. Technology and Culture 22: 512–544.
Hoddeson L. (2008) Bardeen, John. Complete Dictionary of Scientific Biography. Encyclopedia.com. (retrieved December 10, 2014). http://www.encyclopedia.com/doc/1G2-2830905468.html
Hoddeson, L., Braun, E., Teichmann, J., & Weart, S. (eds.) (1991) Out of the Crystal Maze: Chapters from the History of Solid-State Physics. Oxford University Press, New York.
Hoddeson, L., and Daitch, V. (2002) True Genius: The Life and Science of John Bardeen. Joseph Henry Press, Washington, DC.
Riordan, M., and Hoddeson, L. (1997) Crystal Fire: The Birth of the Information Age. W. W. Norton, New York.
Schrieffer R. (1972) Nobel Lecture: Macroscopic Quantum Phenomena from Pairing in Superconductors. Nobelprize.org. Nobel Media AB 2014. Retrieved 10 December 2014. http://www.nobelprize.org/nobel_prizes/physics/laureates/1972/schrieffer-lecture.html