Prof. Dr. William Lawrence Bragg > Research Profile
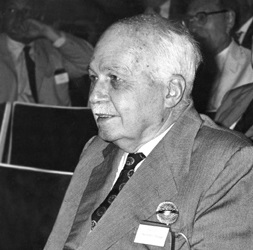
by Luisa Bonolis
William Lawrence Bragg
Nobel Prize in Physics 1914
The Contradictory Nature of X-rays
William Lawrence Bragg was born in Adelaide in 1890, the eldest son of the physicists Sir William Henry Bragg and Lady Gwendoline Bragg, daughter of Sir Charles Todd, Postmaster-General, Superintendent of Telegraphs and Government Astronomer in South Australia. Lawrence Bragg graduated from College in 1905, at the age of fifteen. Only by special arrangement was he allowed to enter the University of Adelaide, where in three years he completed four years of study in mathematics and two years of physics. When his father was offered the Cavendish Professorship of Physics at the University of Leeds in 1908, the family moved to England. In 1909, he was admitted at Trinity College, Cambridge. After one year, at the suggestion of his father, he transferred to physics. After obtaining first class honours in the natural science tripos in 1912, he began doing research under J. J. Thomson at Cambridge.
At that time his father, William Bragg, who conducted research on radioactivity and X rays, became strongly interested in a work published by Walter Friedrich, Paul Knipping and Max von Laue in which they claimed that they had observed the diffraction of X rays by a crystal, similar to the way visible light diffracts through holes, or gratings. Laue's explanation was that the spaces within the regular structure of a crystal were of a size similar to the wavelength of the rays, fulfilling the condition under which diffraction occurs. After Röntgen discovered X-rays in 1895, an achievement for which he was the first physicist to be awarded the Nobel Prize in 1901, many efforts had been done to locate diffraction or interference phenomena in order to solve the question of whether or not they were a form of electromagnetic radiation with a very short wavelength or represented the ejection of any small particles. Röntgen himself used a slit to look for X-ray diffraction. Just like electromagnetic radiation, in particular light in the visible spectrum, the mysterious Röntgen rays exhibited polarization, scattering by matter (already observed by Röntgen himself), and the photoelectric effect. All attempts to display diffraction proved at best inconclusive, though such phenomena were to be expected if X-rays were electromagnetic in nature. From the outset there were supporters of this assumption, and further evidence in support of the wave theory was provided by Charles Barkla, who was later awarded the Nobel Prize in Physics 1917 “for his discovery of the characteristic Röntgen radiation of the elements”. His work, completed in 1906, proved unambiguously that X-rays can be polarized. Notwithstanding these major arguments, the wave theory initially did not meet with complete acceptance.
William Bragg, who had become increasingly engaged in the debate about the nature of the mysterious X-rays, became a firm proponent of the corpuscular view. He wondered how an expanding wave pulse was supposed to energetically ionize an electron in a distant atom and instead suggested that X- and gamma-rays were material particles, proposing that a gamma-ray was simply an alpha-particle and beta-particle electrically bound together to form an uncharged “neutral pair.” Such a pair would have great penetrating power, being unaffected by magnetic and electric fields, ultimately suffering a violent encounter with matter in which the negative particle could emerge as a secondary electron (the photoelectric effect). The same properties, Bragg pointed out, could characterize X-rays. From 1906, he undertook a series of complex experiments that he claimed strongly supported this hypothesis. He became the major British advocate of particulate X-rays, and defended his view in intense discussions in the pages of the journal Nature with Charles Barkla and other supporters of the wave theory. A lively controversy erupted and supporters of the corpuscular view were able to quote a series of phenomena that, in actual fact, remained inaccessible to explanation by the wave theory. While Bragg and other physicists were simultaneously attempting to establish the nature of X-rays and gamma-rays, Einstein was reaching profound insights into the nature of high-frequency black-body radiation and ultra-violet light; however, his light quantum hypothesis would be greeted with deep and prolonged scepticism.
This controversy made Bragg well known and led to his being offered the professorship in Leeds, where he installed a new research laboratory to study the scattering effects of X- and gamma-rays.
X-rays and Crystals
In June 1912, then, news of Laue’s discovery reached William Bragg, who received a letter from a colleague including one of Laue's photographs. Lawrence and his father discussed the German experiment, and William tried to explain the diffraction effect as the shooting of corpuscles down avenues between lines of atoms in crystals. The young Lawrence also set up an experiment in his father's laboratory and tried, unsuccessfully, to explain the Laue spots in term of X-ray particles shooting down the open channels between the crystal atoms. His father, however, was reluctant to abandon his model, seeking instead to find “one theory which possesses the capabilities of both” models.
At the end of the 1912 summer holidays, Lawrence went back to Cambridge as a research student and continued to study with great attention the Laue results and in particular the published photographs. He came to the conclusion that this was indeed a diffraction effect. He abandoned all earlier projects and concentrated fully on the diffraction question. As Lawrence himself explained in his Rutherford Memorial Lecture of 1960, “It must be difficult nowadays to imagine how utterly ignorant physicists were at that time of the geometry of three-dimensional patterns. I happened to know about it, because Pope and Barlow had proposed a valency-volume theory of crystal structure which although incorrect was highly suggestive ... It brought home to me the fact that atoms in space lattices were arranged in planes.” Von Laue's explanation was formulated in terms of crystals viewed as a three-dimensional grid or lattice structure of regularly repeating atoms. However, the problem of calculating the crystal structures from von Laue's formulae was an exceedingly complicated one. What was to be clarified was whether the structure of the crystal and the wavelength of the X-rays had any influence on the diffraction pattern. Not only the space lattices, but also the wavelengths and the intensity-distribution over the various wavelengths in the spectra of the X-rays were unknown quantities. Lawrence Bragg visualized the lattice structure as being constructed from a series of sheets of atoms, laid one on top of another, and conceptualized the observed effect as that of the reflection of X rays by successive parallel crystal planes, acting like mirrors. The intricate pattern of dots that were produced by passing X-rays through a crystal was thus caused by a complex series of interactions in which some X-rays were reflected off the first sheet of atoms encountered, some off the second, some off the third, and so on. In this way, the ratio between the wavelengths and the distances of the planes from each other could be calculated by a simple but powerful equation: nlambda=2dsin(theta). Here n is an integer number, lambda is the wavelength of incident wave, d is the spacing of the planes in the atomic lattice, and theta is the angle between the incident ray and the scattering planes (the glancing angle). This expression became universally known in the community of crystallographers as “Bragg's law.” Lawrence confirmed his suspicions by obtaining specular reflection of X-rays from mica sheets in the laboratory. He could also successfully explain the Laue results by assuming, in addition, that the structure of the zincblende crystal was face-centred cubic rather than simple cubic. He presented his insights to the Cambridge Philosophical Society in early November 1912.
Bragg’s law (and the notion of reflection) became particularly important in the early development of X-ray crystallography, because it rendered the process of diffraction easier to visualize and simplified calculations, allowing analysis of various crystals. Reflection takes place only when Bragg's Law is satisfied, and so the spacing d of the planes parallel to any face under examination can be found by measuring the glancing angle theta. In finding the angle at which a monochromatic beam of X-rays of known wavelength is reflected by the various faces of the crystal, the dimensions of the unit cell of the crystal can be found by measuring d for several faces. Having determined the dimensions of the unit cell, one can calculate how many atoms or molecules are contained within it, using as data the density of the crystal and the mass of each molecule. The second step in analysis consists in the determination of the manner in which the atoms are grouped together to form each unit of the structure. It is here that the principal difficulty is experienced. The structure of the group will influence the strength of the various reflexions in a way similar to the influence exerted by the form of the lines ruled on a grating on the relative intensity of the spectra it yields. Some spectra may be enhanced in intensity, some reduced. The rays are diffracted by the electrons grouped around the centre of each atom. In some directions a strong scattered beam will found. In others their effects almost annul each other by interference. The exact arrangement of the atoms is to be deduced by comparing the strength of the reflexions from different faces and in different orders (that is for different values of the integer n in Bragg’s Law). In their early work, the Braggs made a series of approximate assumptions in order to unravel the complex tangle of information that reflexions from some crystals delivered.
The Birth of X-ray Crystallography
When Lawrence travelled home to Leeds for the Christmas holidays at the end of 1912, the unique father-son collaboration began in earnest. They joined forces embarking on a brilliant period of intense and productive collaboration. In January 1913, William Bragg succeeded in detecting the reflected rays with an ionization chamber. Based on Lawrence's reflection idea, rather than on the notion of diffraction by a three-dimensional grating put forward by Laue, as well as on William Bragg's high level of experimental skill and familiarity with handling X-ray tubes and electroscopes, they managed to construct the first X-ray spectrometer. William adapted a standard optical spectrometer and replaced the light source and focusing tube with an X-ray source and collimator. The chosen crystal replaced the prism or optical grating on the rotatable table in the centre, and a rotating ionization chamber (for detecting the diffracted X-rays) replaced the optical telescope.
Their first joint paper described the spectrometer and related their investigations on a variety of crystals. Groups of peaks in the reflected X-ray intensity as a function of angle were identified as arising from the monochromatic radiations of the X-ray source, a focus of William's interests. While the atomic spacing of a crystal, d, can be determined for X-rays of known wavelength lambda, the wavelength of the X-rays can be determined if a crystal of known d is used, given that the glancing angle is measured.
Initially, William used the spectrometer to investigate the spectral distribution of the X rays, relations between wavelength and Planck's constant, the atomic weight of emitter and absorber, but soon after, according to Lawrence's relation, a known wavelength was used in order to determine d, the distances between the atomic planes, and thus the structure of the crystal mounted in the spectrometer. The great strategic importance of the ionisation spectrometer was that it enabled reflexions to be studied one by one. By means of this device the angles between planes of the crystal structure, and the spacings of the planes could be investigated and measured out in terms of known wavelengths.
The spectrometer was used to analyse the structure of several salts and small molecules, establishing fundamental mathematical relationships between an X-ray diffraction pattern and the dimensional arrangement of atoms in the crystal producing the pattern. The first crystal structure the Braggs solved was rock salt. It was surprising to find that there were no discernible individual molecules of sodium chloride, contrary to what all chemists thought at the time. The crystal was one gigantic latticework, each sodium ion surrounded by six equidistant chlorides, each chloride by six equidistant sodiums. The distinction thus established between molecular compounds (where crystals are made up of molecules) and ionic compounds (where crystals are made up of arrays of ions) was of enormous importance and enabled scientists to achieve a much greater understanding of the behaviour of solutions. A great success was the identification of the structure of diamond, in which each carbon atom is tetrahedrally bonded to its four neighbours in a face-centred cubic lattice array, as chemists had already theorised. The infinite array of carbon atoms, bonded strongly to others in three dimensions, explained the hardness of diamond. Diamond and graphite have the same composition, but their structures make them mechanically, chemically and electronically very different, as later revealed by the structure of graphite.
William continued to have a strong interest in the structure of X-ray spectra, while Lawrence worked hard at the complete solution of a variety of crystal structures. The Braggs turned the X-ray analysis of simple materials into a standard procedure by 1914. That year, the young Lawrence Bragg was elected fellow and lecturer at Trinity College. The work done by father and son between 1912 and 1914 established the basis of the modern science of X-ray crystallography.
In May 1915, the Braggs received the Barnard Gold Medal of the Columbia University, awarded only once every five years “for meritorious service to science.” Earlier recipients had been prominent physicists, such as Lord Rayleigh, William Ramsay, Henri Becquerel, Röntgen and Rutherford. On 14 November of that same year, two telegrams arrived at the family address notifying that they had been awarded the Nobel Prize for Physics 1915. But later they were informed that the award ceremony had been indefinitely adjourned. The war had brought great uncertainty, and even the decisions on the 1914 Nobel Prizes had been deferred to the following year. As hostilities had continued in 1915, it was decided that the Prizes for 1914 and 1915 should now be awarded. Thus it happened that both von Laue and the Braggs received the great news in the same period. Von Laue was awarded the 1914 Nobel Prize for Physics for his breakthrough discovery, while the Braggs, father and son, were jointly awarded the 1915 Prize for Physics, “for their services in the analysis of crystal structure by means of X-rays.” Because of the disruption caused by World War I, the awards ceremony was cancelled. Only in May 1920 were William and Lawrence invited to a ceremony in Stockholm honouring the prize winners for 1918 and 1919 (Max Planck, Johannes Stark and Fritz Haber). During the same ceremony, both the Braggs and Barkla were to be acknowledged, but they did not participate and Lawrence delivered his Nobel Lecture only in September 1922.
In 1919, he had succeeded Ernest Rutherford as Langworthy Professor of Physics at the University of Manchester, where Bragg returned to his investigations of crystal structures by means of X rays after his war activities as technical adviser on the problem of locating the enemy by the sound of artillery fire. For many years he devoted himself to the study of the complex structures exhibited by the silicate family of minerals, revolutionising the science of mineralogy and placing it on a sound scientific basis.
The decade 1920-30 witnessed a great advance in the application of X-ray analysis to more complex crystals, particularly inorganic crystals. It became possible to analyse ever more complex structures. A better understanding of the laws governing diffraction phenomena, and methods of analysis developed by other scientists were applied to the analysis of the structure of a great number of elements, including metals. For the first time the exact arrangement of the atoms in solids became known. It was possible to see how far the atoms are apart and how they are grouped. The importance of this consists in the light it throws on chemical composition and as the knowledge of crystal structure increased, a far deeper insight into the nature of the forces that bind atoms together was reached. The analysis of X-ray diffraction patterns became a powerful tool for mineralogists, metallurgists, ceramists, and other researchers concerned with the atomic structure of materials. The line of research on organic crystals started in the 1920s confirmed the structural models of organic chemistry. X ray crystallography soon informed almost every branch of science, leading to further developments in spectroscopy and solid-state physics and providing a means to understand the structure of highly complex molecules and materials, eventually giving rise to the field of molecular biology.
At the end of World War II, Bragg helped organise the International Union of Crystallography and served as its first president in 1949. In 1948, Acta Crystallographica started publication, providing the X-ray crystallographers with a journal of their own, with which they could be sure of keeping abreast of new developments by finding all the most important work. The Braggs led the field of X-ray crystallography for several decades, encouraging a new generation of researchers, including John Desmond Bernal and William Astbury, both pioneers of molecular biology. In 1934, Bernal was the first crystallographer to obtain clear images of X-ray diffraction by a protein and became the founder of protein crystallography. Among his students were Nobel laureate Dorothy Crowfoot Hodgkin; Rosalind Franklin, who played a major part in the discovery of the structure of DNA by J. D. Watson and Francis Crick; Aaron Klug, who developed crystallographic electron complexes and got the Nobel Prize for Chemistry in 1982; and Max Perutz.
Starting in the late 1930s, Max Perutz had interested Lawrence Bragg in the crystallographic analysis of the complex globular proteins. This research was resumed after the war, and Bragg organised the research effort, found support for the project, and assembled a team to tackle these new problems. In his activity as an outstanding scientific organiser, Bragg promoted the establishment of specific laboratories for the research into the molecular structure of biological systems and encouraged his protégés Max Perutz and John Kendrew in their work on the X-ray crystallographic structure determination for the proteins haemoglobin and myoglobin, a path that would eventually lead them to be jointly awarded the 1962 Nobel Prize for Chemistry.
The Molecular Biology Unit at Cavendish Laboratory in Cambridge led by Lawrence Braggs attracted researchers who felt that the new field of molecular biology had great promise. Among them were Francis Crick and James Watson who proposed in 1953 a double helix structure for the polynucleotide chain configuration of DNA, an achievement for which they shared the 1962 Nobel Prize in Physiology or Medicine with Maurice Wilkins of King's College in London.
From 1954 until his retirement in 1966, Lawrence Bragg was director of the Royal Institution in London, a period during which he was very concerned with science education, like his father William, always trying to convey the excitement of scientific discovery particularly to schoolchildren.
Lawrence Bragg was only 25 years old when he was awarded the Nobel Prize. He is still the youngest recipient ever of the Prize and has been the only Nobel Laureate so far who has celebrated the Golden Jubilee of his award with a Nobel Guest Lecture in Stockholm, which he gave in 1965.
Bibliography
Bragg W. L. (1922) The Diffraction of X-Rays by Crystals. Nobel Lecture, September 6. http://www.nobelprize.org/nobel_prizes/physics/laureates/1915/wl-bragg-lecture.html
Bragg W. L. (1960) The Rutherford Memorial Lecture, 1960. The Development of X-ray Analysis. Proceedings of the Royal Society of London. Series A, Mathematical and PhysicalSciences 262 (1309): 145-158
Hermann A. (2008) Bragg, Sir William Lawrence. Complete Dictionary of Scientific Biography. Vol. 15. Detroit: Charles Scribner's Sons, pp. 61-64. Gale Virtual Reference Library. http://go.galegroup.com/ps/i.do?id=GALE%7CCX2830904839&v=2.1&u=mpi_vb&it=r&p=GVRL&sw=w&asid=37f3da39d655cf9cdfac208c43444959
Jenkin J. (2001) A unique partnership: William and Lawrence Bragg and the 1915 Nobel Prize in Physics. Minerva 39: 373-392
Phillips D. (1979) William Lawrence Bragg. Biographical Memoirs of Fellows of the Royal Society 25: 74-143
Stuewer R. H. (1971) William H. Bragg's Corpuscular Theory of X-Rays and Gamma-Rays. The British Journal for the History of Science 5 (3): 258-281
Wasson, T. (ed.) (1987) Bragg, W. L. In Nobel Prize Winners, H. W. Wilson Company, New York, pp. 137-139