Prof. Dr. Jerome I. Friedman > Research Profile
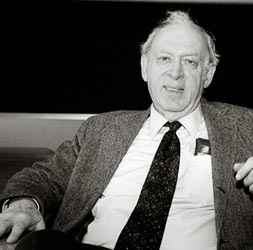
by Roberto Lalli
Jerome I. Friedman
Nobel Prize in Physics 1990together with Henry W. Kendall and Richard E. Taylor "for their pioneering investigations concerning deep inelastic scattering of electrons on protons and bound neutrons, which have been of essential importance for the development of the quark model in particle physics".
Training in Chicago
Jerome I. Friedman was born in Chicago on March 28, 1930, to Jewish parents both emigrated from Russia in the second decade of the 20th century. Initially attracted by artistic disciplines, Friedman developed a strong interest in physics during his high school years, after reading a book by Albert Einstein on the theory of relativity. Upon graduation from high school, Friedman obtained a full scholarship from the University of Chicago to enter the Physics Department in 1950. There, Friedman earned a M.Sc. degree in physics and pursued his PhD studies, which he concluded in 1956. Friedman was particularly stimulated by the teaching style of Enrico Fermi and by the intellectually stimulating encounters with other first rate senior physicists as well as with a great number of talented young colleagues. Friedman’s dissertation research was aimed at investigating the polarization of high-energy protons in elastic and inelastic scattering at the University of Chicago’s synchrocyclotron, employing nuclear emulsion plates as detectors.
After having been discovered in 1937 by the Austrian physicists Marietta Blau and Hertha Wambacher, the nuclear emulsion method was developed by C. F. Powell and his Bristol group to be employed in cosmic ray observations. With the nuclear emulsion method, Powell and his co-workers were able to identify the pion in 1947—a discovery that was fundamental in the understanding of nuclear interactions and in the birth of particle physics in the Post-World War II era.
Friedman was conducting his research under the expert supervision of Fermi, when the latter suddenly died of stomach cancer in 1954.The experimental physicists John Marshall Jr. took the responsibility to supervise Friedman’s project, which was completed by 1956 with the publication of two papers in Physical Review. Thanks to the expertise on nuclear emulsion methodology gained during his doctoral research, Friedman was asked to work in the University of Chicago’s nuclear emulsion laboratory, headed by V. Telegdi. By the early 1957, Friedman and Telegdi confirmed the hypothesis recently advanced by the theoretical physicists C. N. Yang and T. D. Lee about the non-conservation of parity in weak interactions. Employing nuclear emulsion, Friedman and Telegdi observed that parity was indeed violated in the decay chain π+ => µ+=> e+. This experiment confirmed the findings of other groups of experiments that had observed the non-conservation of parity in other weak interaction processes. It helped consolidate the consensus around this experimental discovery, which gained Yang and Lee the Physics Nobel Prize in 1957.
Early work with linear accelerators
In 1957, Friedman moved to Stanford University to join the group headed by Robert Hofstadter at the High Energy Physics Laboratory. Since 1953, Hofstadter had been pursuing a research project on the elastic and inelastic scattering of electrons with the Stanford linear electron accelerator, which by 1955 was able to reach energies around 600 MeV. By analysing the cross sections for the elastic scattering of high-energy electrons, Hofstadter made momentous discoveries concerning the properties of nuclei and their constituents. The most important of them was perhaps that protons and neutrons had a finite size with radius about 10-13 cm and a smooth charge distribution. This meant that nucleons had a structure radically different from that of the electron, which was considered a point-like structureless entity.
Friedman’s work in Hofstadter’s group was important in many respects. In this period, he learned the techniques involved in the scattering of electrons with linear accelerators and in the use of counter devices. Friedman also began working with Henry Kendall, initiating one of the longest collaborations in modern physics.
Friedman participated in a series of experiments on the scattering of electrons on deuterium. These experiments led Friedman to face the problems concerning the relation between signal and background in inelastic electron scattering. To infer the structural properties of the target particles from the scattering cross sections, it was necessary to take into account the energies and momentum that could be carried off by undetected photons radiated by the electrons after colliding with the target particles. In technical terms, it was necessary to calculate the radiative corrections for the inelastically scattered electrons, and Friedman developed a technique to perform this calculation. At this point, Friedman began collaborating with Kendall, who had also put forward a similar technique. In the late 1950s, Friedman and Kendall began applying their method to the experiment on inelastic scattering of electrons on deuterium. The work on radiative correction by Friedman and Kendall would become fundamental in confirming the astonishing results that were obtained in deep inelastic scattering with the 22 GeV linear accelerator at the Stanford Linear Accelerator Center (SLAC) since the late 1960s.
A new accelerator takes the scene
In 1960, Friedman became a faculty member of the Massachusetts Institute of Technology. He worked on a test of Quantum Electrodynamics (QED) performed at the Cambridge Electron Accelerator (CEA), which was the most powerful electron accelerator of the World when it was completed in 1962. Kendall, who had joined Friedman’s group in 1961, also participated in this experiment.
In the meantime, the U.S. Congress had accepted the financial plan to construct at Stanford the World’s greatest linear accelerator. With its two-mile length, the Stanford linear accelerator was planned to reach energies around 22 GeV, opening new perspectives on the study of electron-induced interactions. In 1962, W. K. H. Panofsky and R. B. Neal began directing the construction of the facility baptised SLAC. During the construction of the SLAC, Friedman and Kendall joined the SLAC team in the planning of experiments to be performed with the newly built electron accelerator. They were part of a collaboration of physicists coming from SLAC, MIT, and Caltech, which proposed to employ the linear accelerator to three aims. First, the collaboration planned to measure the elastic electron-proton scattering with an 8 GeV spectrometer. The second aim was to measure the inelastic electron-proton scattering with a 20 GeV spectrometer. Finally, the experimenters planned to compare the cross sections of the scatterings of electrons and positrons on target particles. The focus of the three-group proposal was the extension of Hofstadter’s work to higher energies to explore the structure and the form factor of the nucleons as well as the properties of hadronic resonances.
In May 1967, the facility was ready to start with the first part of the planned research project. Employing liquid hydrogen as detector, the observations confirmed that the electron-proton cross-section rapidly decreased as the scattering angle increased. In other words, the experiments confirmed that the proton had a diffusely extended structure. At this point, the Caltech group decided to end its involvement in this kind of experiments, probably because the Caltech physicists believed that these experiments would not lead to any significant discovery.
Without their Caltech colleagues, the SLAC-MIT physicists led by Taylor (SLAC) and Friedman & Kendall (MIT) began working on inelastic electron-proton scattering in August 1967. Inelastic scattering means that the target particle does not retain its identity after the collision. It was, in principle, possible to detect the hadronic debris resulting from the inelastic electron-proton interaction. However, the first round of experiments focused only on detecting the scattered electrons in order to make a preliminary study of the cross-sections. After data analysis began in the spring of 1968, the investigators understood that the behaviour of the cross sections of the scattered electrons presented unexpected features. The most evident was that at large angles and high energies the cross section of the scattered electrons was much greater than expected. The radiative correction method previously developed by Friedman and Kendall was fundamental in ruling out the possibility that the strange behaviour of inelastic scattered electrons was an artefact of undetected photons radiated by the electrons.
Deep inelastic scattering and the quark hypothesis
The results of the SLAC-MIT deep inelastic electron scattering experiments did not fit with the idea that the proton was a diffusely extended object. It was very hard to understand the meaning of these data and the relations between them and those obtained in elastic scattering. The work of the SLAC field theorist James Bjorken was invaluable in providing a theoretical framework for the interpretation of these empirical findings. Before the experiment was completed, Bjorken had predicted a phenomenon appearing in inelastic electron scattering that is now called the Bjorken scaling. Starting from the sum rules of the current algebra, Bjorken had hypothesised that the two structure functions W1 and W2 of the target particle (more precisely, W1 and the product v W2) would depend on a single variable (ω=2 Mv/q2, where M is the mass of the target hadron, v energy lost by the electron, and q2 is the squared four-momentum transfer) for large q2 and v (now called the Bjorken limit). The experimenters followed Bjorken’s suggestion to plot the data against the variable ω, and they just found what Bjorken had predicted. The implications of these findings, however, were far from being clear.
Several different theoretical approaches were put forward to explain the result of the deep inelastic scattering experiments, i.e., the rather weak dependence of the cross section on q2 and the Bjorken scaling. Schematically, there were two kinds of approaches. The first, mainly championed by Feynman, was called the parton model. According to this model hadrons were clouds of constituent particles (partons), which act as quasi-free particles in high-energy interactions, i.e., they did not interact with one another while the virtual boson mediating the scattering was exchanged. Several hypotheses were advanced as for the nature of these hypothetical partons. Some physicists proposed that the partons were the spin ½ fractionally charged quarks—introduced by M. Gell-Mann and, independently, by G. Zweig in 1964 to explain the symmetries of the hadrons that were being discovered. Some theorists supposed, instead, that partons were other kinds of particles with different charge and spin, e.g. pions and bare nucleons.
The second approach included a series of models, often referred to as non-constituent models, which did not interpret the results of the SLAC-MIT experiment as a consequence of the collision of electrons and point-like constituents of the nucleon. The most successful of these models was perhaps the Vector Dominance Model, which explained the deep inelastic scattering results as consequence of the hypothetical coupling between photons and ρ mesons.
From 1969 onward, Friedman, Kendall, Taylor and their co-workers performed a long series of experiments on deep inelastic electron scattering aimed at testing the predictions of the several theoretical models—an endeavour that lasted till 1975. Experiments on deep inelastic electron scattering over a large range of angles and energies rapidly ruled out the non-constituent models. As for the nature of the partons, it was necessary to compare some specific predictions of the parton models that depended on the charge and spin of the hypothetical partons.
Theorists found that a combination of the structure functions W1 and W2—denoted R—depended in a predictable way on the partons’ spin. In the Bjorken limit, R was calculated to be zero in case partons had spin 1/2, but non-zero for values of spins 0 or 1. In 1971, after an extensive set of observations over an ample range of energies and four-momentum transfers, the experimenters concluded that R was very small and, in view of possible experimental errors, consistent with the hypothesis of partons as spin ½ particles.
The decisive experiments in the clarification of the nature of partons were those on the inelastic scattering of electron on neutrons. While the earlier experiment employed liquid hydrogen as target, since 1970 the SLAC-MIT teams began using also liquid deuterium to compare the structures of two different hadrons. By measuring the ratio of neutron and proton cross-sections, the experimenters found that the result were fairly consistent with the hypothesis put forward by the MIT theorists V. Weisskopf and J. Kuti that the proton and the neutron were made of three quarks (uud and ddu, respectively) and neutral gluons, which do not interact with electrons and carried one half of nucleons’ momentum.
Even though by 1972 the experimental results seemed to confirm the Weisskopf-Kuti proposal, there was still much uncertainty, and the experimenters were very cautious on inferring the implications of their findings. One of the central problems was that fractionally charged particles had never been observed. If quarks were the constituents of nucleons they should be permanently confined within the hadrons. However, permanent confinement was hardly compatible with the property of the parton model according to which partons behave as free particles during high-energy interactions. The discovery of D. Gross and Wilczek that the gauge theory of quarks and gluons had the property of asymptotic freedom rapidly changed the views of many physics practitioners. Asymptotic freedom was just the property needed to explain the Bjorken scaling, and from that moment onward the SLAC-MIT experiment was considered as the first empirical evidence of the quark structure of hadrons.
Calculations made in the framework of the gauge field theory of strong interactions (called Quantum Chromodynamics) predicted a logarithmic deviation from scaling that was also consistent with the results of the experiments Friedman and his co-workers were carrying on at SLAC.
Further confirmation came from different experiments performed at the Fermilab and, especially, CERN, on deep inelastic neutrino scattering. When the CERN team made the preliminary announcement that the neutrino cross-section confirmed the phenomenon of scaling in the summer of 1973, the physics community was convinced that the quark picture had received substantial empirical confirmation. Other theoretical developments and empirical confirmations led Quantum Chromodynamics to become one of the two pillars of the Standard Model of particle physics by the end of the 1970s. In1990, Friedman, Kendall and Taylor, were awarded the Nobel Prize in Physics, “for their pioneering investigations concerning deep inelastic scattering of electrons on protons and bound neutrons, which have been of essential importance for the development of the quark model in particle physics.”
Testing the prediction of the Standard Model with scattering experiments
In the period between 1967 and 1975, Friedman was completely involved in the electron scattering experiments at SLAC and the analysis of the data. Before this long-lasting research project came to an end, Friedman began collaborating with other institutions on other experiments to be conducted at Meson Laboratory, housed by the recently established Fermilab. Friedman worked on the design of a beam line and single-arm spectrometer. With this apparatus, Friedman and his co-workers performed a series of experiments aimed at testing various properties of the elementary particles in elastic and inelastic scattering of the type hadron-hadron. Later, Friedman, Kendall, and their group joined a different project on the construction of a large neutrino detector at the Fermilab. With this detector, the collaboration of experimenters investigated the scattering of neutrinos (or anti-neutrinos) on nucleons. The result of the large series of experiments performed in the early 1980s, confirmed the prediction of the Standard Model concerning the weak neutral currents. In the 1980s, Friedman was occupied by administrative duties as Director of the Laboratory for Nuclear Science at MIT (from 1980) and as Head of the MIT Physics Department (1983-1988). Friedman managed to occupy important roles in the development of accelerator machines in the United States. Before the 1990s, his MIT group made important contributions to the construction of the Large Detector for electron-positron annihilation at the Stanford Linear Collider. This detector was especially designed to detect Z bosons and operated from 1992 to 1998. His MIT group was also called to construct a detector for the Super Conducting Supercollider—a large proton collider that was planned to become the premier World’s facility for the investigation of particle interactions. In 1993, after six years of work the project was eventually cancelled by the U.S. Congress that did not confirm the funding of this 8-billion dollar operation. The dismissal of the Superconducting Super Collider was perhaps the most dramatic stroke to the American high-energy physics community, which now relies on the research done at the 14 TeV CERN Large Hadron Collider to open a window on the unexplored properties of the microworld at higher energies.
Bibliography
Fitch V. L., & Rosner L. (1995) Elementary Particle Physics in the Second Half of the Twentieth Century. In Brown, L., Pippard, B., & Pais, A. (Eds.). Twentieth century physics (Vol. 2). AIP, New York, pp. 635-794.
Friedman, J. I. (1991) Jerome I. Friedman - Biographical". Nobelprize.org. Nobel Media AB 2014. Accessed 9 July 2014.
http://www.nobelprize.org/nobel_prizes/physics/laureates/1990/friedman-bio.html
Friedman, J. I. (1991) Deep Inelastic Scattering: Comparisons with the Quark Model. Reviews of Modern Physics, 63(3), pp. 615-627.
Friedman, J. I., & Kendall, H. W. (1972) Deep Inelastic Electron Scattering. Annual Review of Nuclear Science, 22(1), pp. 203-254.
Galison, P. (1997) Image and Logic: A Material Culture of Microphysics. University of Chicago Press, Chicago.
Hoddeson, L. Brown, L. M., Riordan, M. & Dresden, M., (1997) The Rise of the Standard Model: Particle Physics in the 1960s and the 1970s. Cambridge University Press, Cambridge.
Kendall, H. W. (1991) Deep Inelastic Scattering: Experiments on the Proton and the Observation of Scaling. Reviews of Modern Physics, 63(3), pp. 597-614.
Pais, A. (1986) Inward Bound Of Matter And Forces In The Physical World. Clarendon Press, Oxford.
Pickering, A. (1999) Constructing Quarks: A Sociological History of Particle Physics. University of Chicago Press, Chicago.
Taylor, R. E. (1991) Deep Inelastic Scattering: The Early Years. Reviews of Modern Physics, 63(3), 573-595.