Black Holes
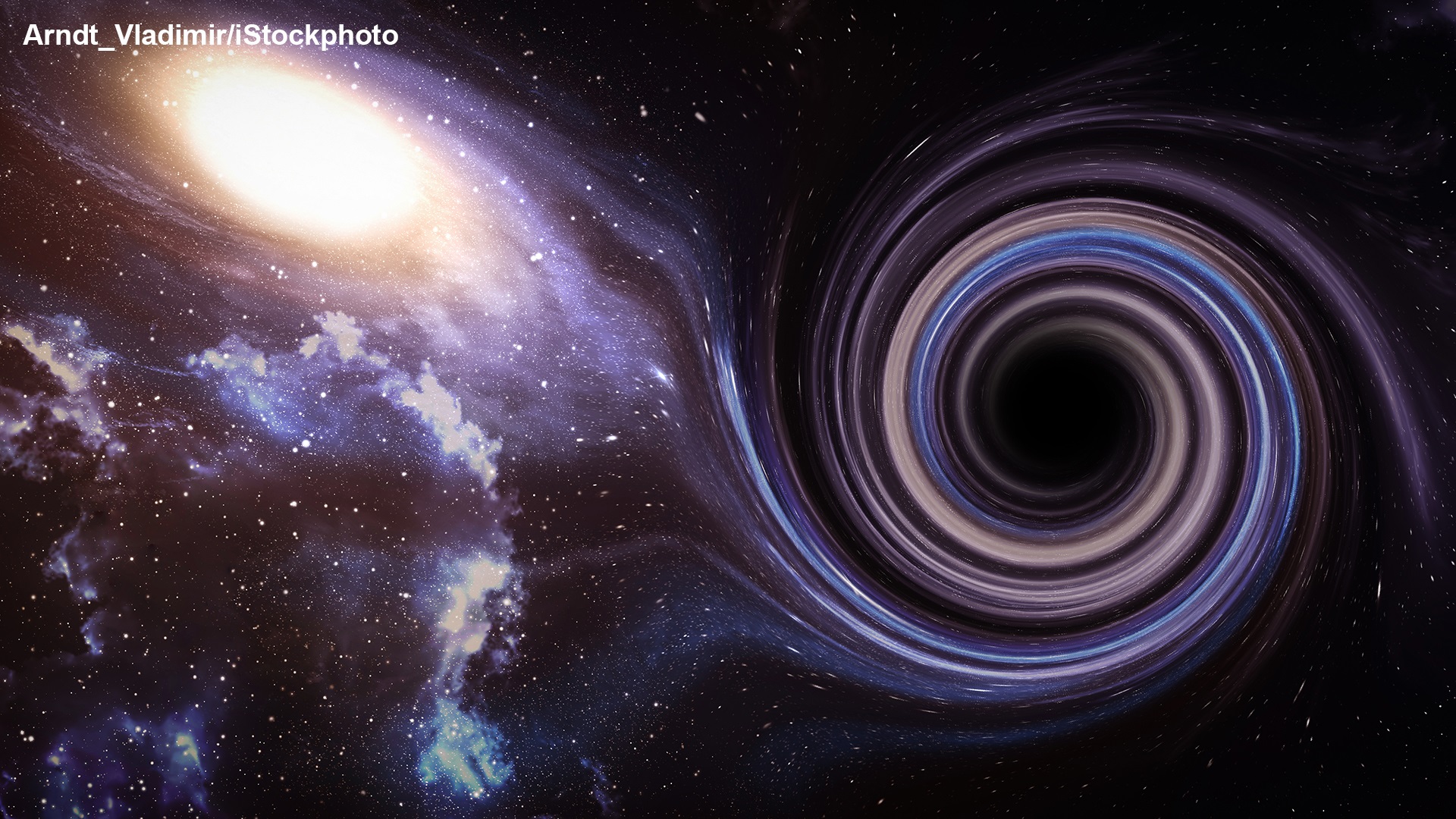
by Ben Skuse
Black holes. They inspire awe and fear in equal measure. Like cosmic monsters, they greedily feed on anything unfortunate enough to cross their path. What’s worse, they hide in plain sight, only visible through their gargantuan gravitational effects and the lucky few bright jets of energy and matter that are blown far out into space just moments before capture.
In a black hole, matter becomes so densely packed that its gravity lets nothing escape from its clutches. It is an object whose edge is where time comes to a stop and whose centre may hide a point of infinitely small volume and infinite density – a place where the laws of physics break down.
Because of these mind-bending properties, for decades, black holes were regarded as little more than an aberration – a stain on Albert Einstein’s (1921 Nobel Prize in Physics) otherwise perfect description of the macroscopic physics of the universe: his general theory of relativity.
(00:08:16 - 00:16:04)
The complete video is available here.
From Space Aberrations to the Theory of Singularity
Karl Schwarzchild’s 1916 solution to Einstein’s equations – penned while serving in the German army during World War I – showed that if matter can be drawn tightly enough together, it can suffer a cataclysmic collapse to an infinitesimal point called a singularity. But the scientific community simply wasn’t buying it. In 1935, Arthur Eddington summed up many physicists’ thoughts on the subject: “There should be a law of nature to prevent a star from behaving in this absurd way”.
It has taken some 60 years of concerted theoretical and experimental effort for scientific opinion to finally swing fully in favour of black holes’ existence. For example, in a 2021 Lindau panel discussion, Rainer Weiss (2017 Nobel Prize in Physics) described how pockets of resistance to black holes still existed at the Massachusetts Institute of Technology (MIT) right up to the discovery of the first black hole merger in 2015.
(00:06:41 - 00:07:30)
The complete video is available here.
And in fact, so recent has been this swing that the 2020 Nobel Prize in Physics was the first given specifically for unravelling the mysteries of black holes. Mathematical physicist Roger Penrose received half the Prize “for the discovery that black hole formation is a robust prediction of the general theory of relativity.” The other half was jointly awarded to astrophysicists Reinhard Genzel and Andrea Ghez “for the discovery of a supermassive compact object at the centre of our galaxy” that is strongly believed to be a black hole.
With the freedom to choose their lecture topics at the Lindau Meetings, over the years some Nobel Prize recipients have been more definitive about the existence of black holes than the Nobel Committee and other members of the physics community. As early as 1976, Antony Hewish (1974 Nobel Prize in Physics) was already discussing exciting developments in black hole physics.
(00:03:00 - 00:04:55)
The complete video is available here.
Hewish’s talk gives an insight into the confidence some members of the community had, that black holes were a real part of the universe by that time. This confidence was born from both theoretical and observational breakthroughs, not least a decade of discovery that had just come to an end; a decade dubbed the Golden Age of Black Hole Physics.
The Golden Age of Black Holes
Ushering in this Golden Age was New Zealand mathematician Roy Kerr. In 1963, he found a solution to Einstein's equations that describes the space outside a rotating star or black hole. Before his work, many in the community believed that perturbations caused by rotation would stop black hole horizons ever forming. His contribution silenced these critics, catapulting black holes from interesting theoretical concepts to realistic prospects. Given all stars rotate, Kerr’s solution provided a realistic representation of black holes, suggesting untold numbers may be hiding in the universe.
Just two years later, in 1965, Penrose published his seminal paper establishing further key black hole concepts in the real world. Regarded as the first genuine post-Einsteinian result in general relativity, in the paper Penrose introduced the notion of a ‘trapped surface’ – a closed two-dimensional surface that only allows light rays to converge toward the centre.
Up to then, all attempts to represent black holes from Einstein’s equations required black holes to exhibit some kind of symmetry, even Kerr’s solution. By assuming all surfaces within the black hole are trapped surfaces, Penrose removed this requirement. Black holes could form in the messy unsymmetric real world. An imploding star will necessarily produce a singularity once it has formed an event horizon.
Following on from these breakthroughs, intense work characterising black holes eventually culminated in 1973 with James M. Bardeen, Brandon Carter and Stephen Hawking producing an analogy to the four laws of thermodynamics, but for black holes. Much of the mystery surrounding black holes had been removed, at least theoretically.
(00:04:53 - 00:07:38)
The complete video is available here.
Seeing Into the Dark – The Advent of X-Ray Astronomy
At the same time as theorists like Kerr, Penrose and Hawking were proving that black holes were a realistic prediction of general relativity, astronomers were starting to find real-world evidence of black holes’ existence in the cosmos.
In 1964, Cygnus X-1 was discovered. Using X-ray instruments carried aboard sounding rockets – to get above the obscuring effects of Earth’s atmosphere – Cygnus-X1 was revealed to be one of the strongest X-ray sources in the night sky. Improved observations from the first X-ray astronomy satellite, NASA’s Uhuru, in 1970 illuminated the pulsating nature of Cygnus-X1, with the X-ray light from the area fluctuating several times a second.
Further observations exposed Cygnus-X1 as not one object but two – a supergiant star, incapable of emitting the bright X-rays alone, and a hidden massive and compact companion. This mysterious companion was estimated to be 15 times the mass of the Sun, far exceeding any theoretical limit for white dwarfs or neutron stars. By the end of the Golden Age of Black Hole Physics in 1973, most researchers in the astronomical community had conceded that Cygnus X-1 was most likely a stellar-mass black hole.
It is now known that the X-ray light from Cygnus-X1 comes from the black hole’s accretion disc: a flattened band of gas, dust and other debris from its nearby companion star. The particles within the accretion disc are accelerated to tremendous speeds by the black hole’s gravity, in the process releasing heat, and very bright X-rays and gamma rays out into the universe which can be seen by X-ray detectors. By also measuring the orbit of the star around the hidden object, scientists have inferred its mass and size precisely, and thereby confirmed it is indeed a black hole. In the years following Cygnus-X1’s discovery and characterisation tens of further stellar-mass black holes in the Milky Way have been uncovered in a similar way.
Cygnus-X1’s discovery and characterisation, and that of many more black holes, would not have been possible without the advent of X-ray astronomy, pioneered by Riccardo Giacconi (2002 Nobel Prize in Physics). Giacconi worked on the first sounding-rocket X-ray detectors in the 1950s and 60s, and he also proposed and developed Uhuru and later X-ray satellites including Chandra.
In his 2004 Lindau talk, Giacconi described not only X-ray astronomy’s key role in elucidating the nature of Cygnus-X1 and other stellar-mass black holes, but also its power to probe the cosmos for different but equally awe-inspiring and mysterious objects – supermassive black holes.
(00:07:46 - 00:08:50)
The complete video is available here.
(00:09:27 - 00:12:09)
The complete video is available here.
Our Own Black Hole – At the Heart of the Milky Way
Supermassive black holes are millions to billions of times the mass of the Sun and are nowadays thought to reside at the heart of many galaxies, with thousands of candidates discovered and dozens having been observed and characterised. If that’s the case, does our galaxy, the Milky Way, harbour one?
In 1974, astronomers Bruce Balick and Robert L. Brown discovered a powerful radio source in the Galactic centre that emits synchrotron radiation that they named Sagittarius A*. Intrigued, Charles H. Townes (1964 Nobel Prize in Physics) developed a novel infrared detector incorporating a precision CO2 laser to study this region in more depth.
By the mid-1980s, Townes and his then doctoral student Reinhard Genzel had found evidence for a huge mass in the region – a strong hint of a supermassive black hole, but not proof. From the 1990s onwards, Genzel’s team and that of his US contemporary (and Nobel co-recipient) Andrea Ghez began to peer into the heart of the Milky Way with more advanced instruments to look for more conclusive signatures of this black hole. They reasoned that the stars near such an extreme object would have tell-tale orbits, accelerating as they fell closer and closer to the centre.
With Genzel using the four 8 metre telescopes of the European Southern Observatory in Chile, and Ghez the Keck telescopes on Mauna Kea in Hawaii, by 2008/9 they had their proof. The trajectories of several close-in stars indicated that Sagittarius A* measures less than 125 times the distance between Earth and the Sun, even though it contains 4 million solar masses.
In his 2021 Lindau lecture, Genzel described his '40-year journey' to providing firm evidence of the existence of the Milky Way’s supermassive black hole.
(00:17:04 - 00:26:15)
The complete video is available here.
During the same meeting, Genzel also discussed how he and his colleagues are now using Sagittarius A* as a general relativity laboratory, just as Subrahmanyan Chandrasekhar (1983 Nobel Prize in Physics) predicted when he shared his thoughts on why black holes make a perfect testing ground for Einstein’s theory in his 1988 lecture.
(00:03:52 - 00:06:13)
The complete video is available here.
(00:30:50 - 00:36:54)
The complete video is available here.
New Eyes on the Universe – Detecting the Reverberations of Black Hole Collisions
Most recently, astronomers have been using a completely different technique for detecting black holes, and to dazzling effect. On 14 September 2015, the Laser Interferometer Gravitational-Wave Observatory (LIGO) and Virgo collaborations made the first direct observation of gravitational waves.
These cosmic ripples in the fabric of spacetime were predicted by Einstein’s equations 100 years earlier, but their existence in reality had been hotly debated ever since. Indeed, Paul A. M. Dirac’s 1959 lecture was dedicated to showing the audience why they should regard gravitational waves as having “physical significance”.
(00:20:59 - 00:21:27)
The complete video is available here.
It may have taken over 60 years, but Dirac was proved right. Dubbed GW150914 (from "Gravitational Wave" and the date of observation 2015-09-14), the detected signal came from the collision of two black holes 1.3 billion years ago, which for about 1/10 of a second produced ripples of distorted space with a power 50 times larger than that of all the stars in the universe combined before flowing out into the cosmos.
George F. Smoot (2006 Nobel Prize in Physics) was the first to report on the detection in Lindau in 2016.
(00:01:00 - 00:04:23)
The complete video is available here.
Soon after their 2017 Nobel Prize in Physics award, the key contributors to the first gravitational wave detection – Rainer Weiss, Barry C. Barish and Kip S. Thorne – flocked to Lindau.
After a detailed history of the long road to gravitational waves by Joseph H. Taylor Jr. (1993 Nobel Prize in Physics) in 2019, Rainer Weiss was the first of the three Laureates to lecture at Lindau, providing an overview of the field, including an interesting perspective on why it took so long for gravitational waves to be detected.
(00:00:20 - 00:05:03)
The complete video is available here.
(00:00:15 - 00:01:25)
The complete video is available here.
Two years later, the Lindau audience was treated to consecutive lectures from Barish and Thorne. Barish’s talk introduced LIGO, the vast experiment consisting of two large L-shaped observatories in the US that use mirrors spaced four kilometres apart to detect tiny stretches and compressions of spacetime of the order of one thousandth the size of a proton.
(00:18:32 - 00:25:30)
The complete video is available here.
Immediately following Barish, Thorne presented the first observation itself, revealing how well it matched theory, and even showing the audience a brief visualisation of how the black hole merger warped spacetime and created gravitational waves.
(00:12:55 - 00:16:12)
The complete video is available here.
With over 50 gravitational-wave signals from the merging of pairs of black holes and of pairs of neutron stars now reported, gravitational wave astronomy has established itself as a new and exciting probe of black holes and general relativity. Upgrades to existing facilities are ongoing and new gravitational wave observatories are being built. There is even the promise of a gravitational wave detector in space. With traditional observational techniques improving to the point where we can now actually see the radio emission from a supermassive black hole in another galaxy, might all of this mean we have entered a new Golden Age of Black Hole Physics?
The answer from Thorne and many other Nobel speakers is a resounding yes. “Think back to the huge revolution in our understanding of the universe from electromagnetic astronomy by itself over the last several centuries,” Thorne said during his 2021 lecture. “I invite you to speculate what may happen with gravitational wave astronomy and multi-messenger astronomy over the next several decades and centuries, because it's going to be very, very exciting.”
(00:35:33 - 00:38:04)
The complete video is available here.