Nuclear Magnetic Resonance
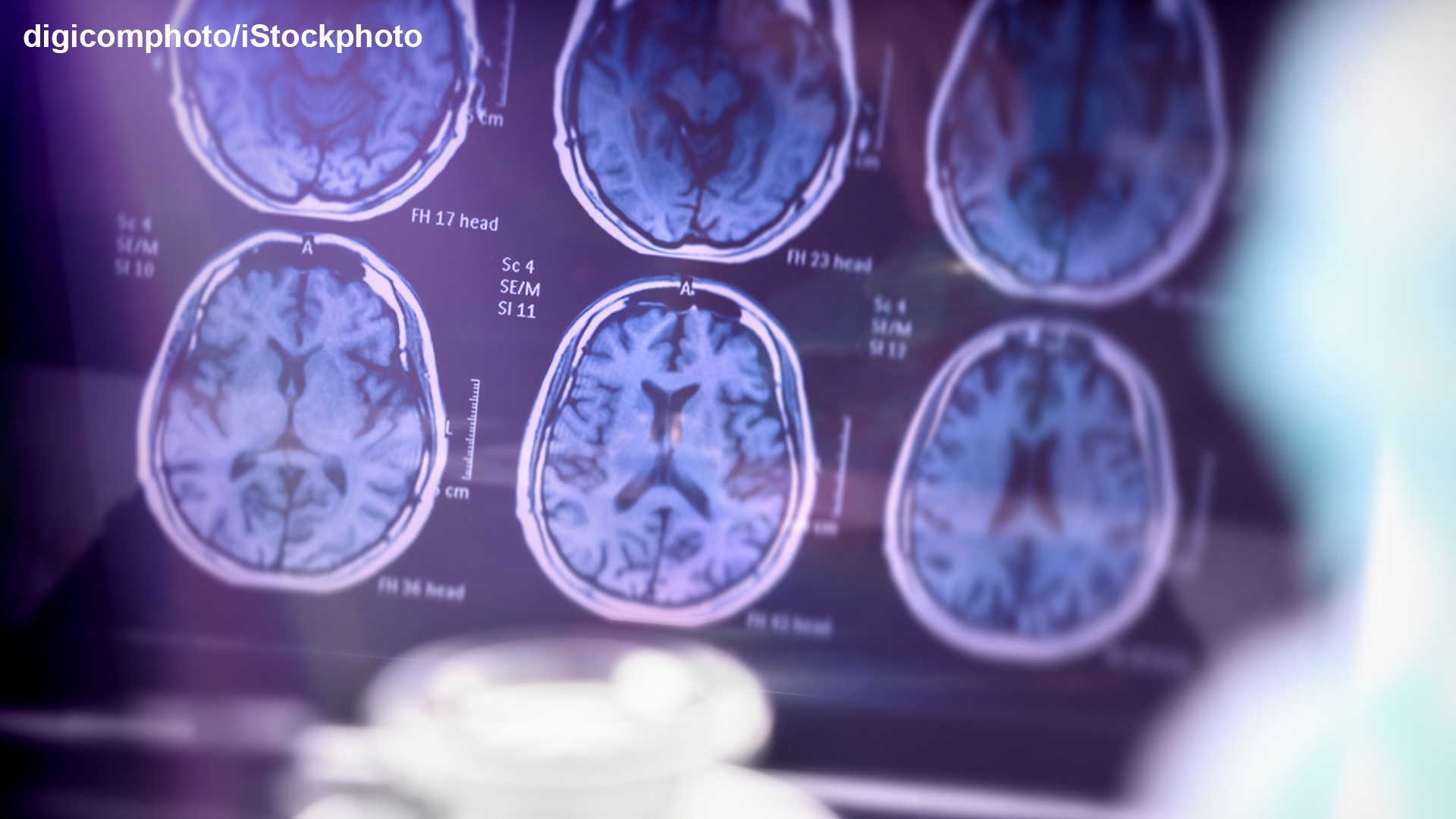
by Joachim Pietzsch
Introducing nuclear magnetic resonance (NMR) means talking about the music of matter and how listening to it makes the score of nature more intelligible. “Each atom is like a subtle and refined instrument, playing its own faint, magnetic melody, inaudible to human ears. By your methods, this music has been made perceptible, and the characteristic melody of an atom can be used as an identification signal“, Harald Cramér, member of the Royal Swedish Academy of Sciences, praised Felix Bloch and Edward Purcell on occasion of the Nobel Banquet in 1952. In similar words, Richard Ernst explained the NMR method forty years later to his audience in Lindau:
(00:22:30 - 00:22:50)
Bloch, Ernst and Purcell are three of six scientists, who received a Nobel Prize explicitly linked to the technique of nuclear magnetic resonance. Bloch and Purcell shared the Nobel Prize in Physics 1952 “for their development of new methods for nuclear magnetic precision measurements and discoveries in connection therewith”. Richard Ernst was awarded with the Nobel Prize in Chemistry 1991 “for his contributions to the development of the methodology of high resolution nuclear magnetic (NMR) spectroscopy”. Kurt Wüthrich received a Nobel Prize in Chemistry 2002 “for his development of nuclear magnetic resonance spectroscopy for determining the three-dimensional structure of biological macromolecules in solution”. Paul Lauterbur and Sir Peter Mansfield shared the Nobel Prize in Physiology or Medicine 2003 “for their discoveries concerning magnetic resonance imaging”. Two laureates in physics, two in chemistry and two in physiology or medicine – the history of NMR seems to span in equal measure all three scientific Nobel disciplines. This impression, however, is misleading, because the invention of NMR has its sole and strong foundation in a basic discovery of physics, as Kurt Wüthrich suggested in his Lindau lecture in 2014.
(00:08:06 - 00:08:56)
Caught in Quantization
Without Otto Stern’s discovery of space quantization and his first direct measurement of the magnetic moment of an atom and without the advancement of this discovery by Isidor Isaac Rabi, who first established radio contact with nuclear magnetic moments, NMR could not have been invented. With good cause, the former was awarded with the Nobel Prize in Physics 1943, the latter with the Nobel Prize in Physics 1944. While Stern only attended the 1968 Lindau meeting and gave no lecture, Rabi attended the Lindau meeting six times between 1956 and 1979. His lecture from 1971 is mainly a reverence for Stern. “The reason I am giving this talk about 60 years of molecular beams”, Rabi said, “is to a great degree in remembrance of this very great German physicist, who unfortunately had to leave his country and its culture and went to America where he never did succeed in entering a new culture and I regard it as a great personal tragedy for this great man, and an enormous loss for science.” Otto Stern made his discovery in February 1922 together with his colleague Walther Gerlach in Frankfurt/Main, whose university had been founded just eight years before in the spirit of the city’s liberal bourgeois tradition. At that time, in the early years of the Weimar Republic, it was “the only German university where republican professors were not outnumbered by conservatives pining for the old order; its charter outlawed all racial and religious discriminations”[1]. Besides Berlin, Frankfurt therefore was an especially thriving hub of science and the humanities then and a perfect place for a creative and fruitful German-Jewish symbiosis. Before we describe the Stern-Gerlach experiment in more detail, let us listen to Isaac Rabi’s account of it:
(00:07:26 - 00:13:29)
A Triumph of Experimental Physics
From today’s perspective, the experiment that Stern and Gerlach conducted appears to be easy. Yet in 1922, it was technically extremely challenging. Its successful performance, which took them one year of planning and preparation, was a triumph of experimental physics. Its intention was to find out how atomic magnets behave in a magnetic field. Classical physics and the only just emerging quantum theory gave contradictory answers in this respect. Within a highly evacuated apparatus (first challenge) in which huge temperature differences had to be maintained over many hours (second challenge), Stern and Gerlach evaporated silver in a hot oven and produced a collimated, very thin beam of silver atoms (third challenge) that they sent through a very inhomogeneous magnetic field (fourth challenge) towards a collector plate on which the meager film of atoms had to be made visible to detect their deflection in the magnetic field (fifth challenge). There they observed two distinct lines. The silver atoms had split into two beams. This “was a shock from the moment it was discovered (although it was expected theoretically)”[2]. According to classical physics, the silver beam should have broadened to one large spot because of the random distribution of the magnetic moments of its atoms. Yet obviously the atoms had the choice between two directions only, one parallel and the other anti-parallel to the external magnetic field. Their orientation in space is quantized between two distinct states. The correct explanation for this behavior was given in 1925 by de Laer Kronig, Uhlenbeck and Goldsmith: Every electron possesses an intrinsic spin with a related magnetic moment. In case of silver with its 47 electrons, 46 electrons possess pair-wise opposite spins that cancel each other out. “The forty-seventh unpaired electron also has zero orbital angular momentum, so the only source of magnetic moment is the intrinsic spin, a purely quantum mechanical effect, having no classical analogue. The beam of silver atoms divides in two, depending on the spin of the forty-seventh electron, so there are two possible states of spin which came to be known as up and down.“[3]
With their experiment, Stern and Gerlach did not only lay the foundation for nuclear magnetic resonance. Their “key concept of sorting quantum states via space quantization” resulted in “the prototypes for ... optical pumping, the laser, and atomic clocks, as well as incisive discoveries such as the Lamb shift and the anomalous increment in the magnetic moment of the electron, which launched quantum electrodynamics. The means to probe nuclei, proteins, and galaxies; image bodies and brains; perform eye surgery; read music or data from compact disks; and scan bar codes on grocery packages or DNA base pairs in the human genome all stem from exploiting transitions between space-quantized quantum states.“[4]
The Stern-Gerlach experiment was not mentioned in the quotation of Stern’s Nobel Prize, but E. Hulthén highlighted it in the broadcasted award ceremony speech on 10th December 1944. Stern earned his Nobel Prize “for his contribution to the development of the molecular ray method and his discovery of the magnetic moment of the proton”. Isidor Isaac Rabi in Lindau explained the importance of the latter and its surprising result:
(00:20:25 - 00:22:10)
Isaac Rabi had begun to study physics at Columbia University in 1923. Yet in terms of modern physics, the US was a developing country at that time. “The only people who had read papers on quantum mechanics were the graduate students, but none of the professors”, Rabi remembered in Lindau. So he gave a departmental seminar on the Stern-Gerlach experiment for his fellow students on his own[5]. After he had obtained his Ph.D. in 1927, he set out for a two-year postgraduate journey through Switzerland, Germany and Denmark. “I felt I had to come to Europe, which was the source of all this excitement and inspiration”. He visited Schrödinger, Sommerfeld, Bohr and Heisenberg, but spent the majority of his time in Otto Stern’s lab, who was then working together with Wolfgang Pauli in Hamburg. Practicing the molecular beam method there paved his way to the invention of magnetic resonance almost ten years later at Columbia where he had become a professor in 1929 on the recommendation of Werner Heisenberg.
Radio Waves Cause Quantum Jumps
Stern’s experiments had proven the existence of quantized intrinsic magnetic spins in electrons and nuclei. Although this metaphor does not truly match the quantum mechanical reality, these spins can be compared to tiny gyroscopes precessing with a certain frequency around the direction of an external magnetic field. But is there a possibility to accurately measure the magnetic spins within a hydrogen atom when the strength of the external magnetic field is known? This was Rabi’s fundamental question. With modest distinction, he only briefly eluded on how he solved it in his Lindau lecture:
(00:33:40 - 00:35:45)
Rabi and his collaborators exposed the molecular beam that passes the magnetic field to radio waves. For this purpose, they inserted a loop of wire into the magnetic field and attached it to an oscillating circuit. Then they tuned the frequency of the electromagnetic waves generated by this circuit – until it matched the precession frequency of the nuclei under investigation. In this moment, the resonance phenomenon occurs: The nuclei flip over from one quantum state to the other. Their chance to reach the detector sharply decreases and the detector registers this effect as a marked minimum. The invention of this “resonance method for recording the magnetic properties of atomic nuclei”, as the quotation says, earned Rabi the Nobel Prize in Physics 1944. Based on the artificial laboratory setting of molecular beams as it was, however, the method was not yet applicable for the structural elucidation of molecules.
Introducing Nuclear Induction
Felix Bloch and Edward Purcell developed Rabi’s concept further by complementing it with the principle of nuclear induction. When the radio waves that have caused the quantum jumps in hydrogen nuclei are switched off, the energized nuclei relax back to their initial state and fire out radio signals themselves, which induce an oscillating voltage in a pick-up coil. Bloch and Purcell succeeded in converting these signals into spectra where the radio signals transmitted from each nucleus register as a series of specific peaks and thus can reveal the structure of small molecules: Nuclear magnetic resonance spectroscopy was born. Felix Bloch attended the Lindau meeting twice. The only lecture he gave there in 1976 did not deal with his Nobel Prize winning topic but rather with current aspects of superfluidity. To deepen our understanding of nuclear magnetic resonance, it’s therefore well worth listening to Kurt Wüthrich’s concise explanation:
(00:09:05 - 00:11:49)
An Esoteric Tool Goes High-Tech
In its early years, NMR spectroscopy was still more of an esoteric tool than the method of choice to solve complicated chemical structures. The first commercial NMR spectrometers in the 1950s enabled frequency degeneracy between the two eigenstates of hydrogen nuclei of just 30 Megahertz. Their magnetic field was too weak to provide sufficiently resolved spectra. The radio signals sent from the resonating nuclei remained relatively feeble, and so it was extremely difficult for an experimental observer to discriminate signals from noise. Small amounts of nuclei were almost impossible to detect. Three developments helped to overcome this initial weakness of NMR spectroscopy: One was the rapid improvement in the application of superconductivity. It had been discovered already in 1911 by Heike Kamerlingh Onnes (Nobel Prize in Physics 1913), but began to yield its first practical results only more than four decades later. Several Nobel Prizes in Physics (e.g. 1972, 1973, 1987 and 2003) substantiate the progress in this field. Superconducting magnet coils helped to enhance the field strength of NMR spectrometers dramatically. Today, the first one-billion-hertz machines are in use. They can generate a magnetic field of 23.5 Tesla. The other development, as in science in general, was the exponential growth of computing power. The third development was a sharp increase in detection speed and sensitivity, which led to the advent of high-resolution NMR and mainly was the merit of Richard Ernst.
After receiving his PhD in the early 1960s, he joined the Californian company Varian Associates. There, Ernst was excited by his boss Weston Anderson's work on a mechanical device called the "wheel of fortune". Instead of the usual method of bathing a compound in a slowly tuned sweep of radio waves, Anderson was trying to develop a mechanical generator that excited atomic nuclei with more than one radio frequency, which he hoped would save time by plotting several signals in parallel. This approach eventually proved to be unpractical, but Anderson suggested that Ernst work on another possibility for acquiring parallel data. And indeed Ernst achieved a similar effect by using a series of short and intense radio pulses. He plotted all the signals together as a function of time after each pulse. A computer converted this complex graph into the conventional NMR pattern, using the same mathematical calculation that is used to identify molecular structures in X-ray crystallography, a formula called the Fourier transformation (FT).
(00:17:45 - 00:19:44)
Ernst likened the way in which the new process called Fourier Transform NMR, or FT NMR, identifies nuclei to listening to a piano. "Imagine you want to find out which strings are broken in an old piano, and how time consuming it would be to strike one key after the other," said Ernst. "FT NMR is like striking all 88 keys at once and immediately identifying which keys are still functioning."[6] This new method „could increase the sensitivity by a factor of 30 or more“[7]. In addition, it became possible now to detect not only hydrogen signals but also those of 13C and 15N-isotopes (NMR-active nuclei need to have an odd number of protons and neutrons). In his Lindau lecture from 2006, Ernst explained the general importance of the Fourier transformation in science:
(00:03:22 - 00:06:57)
From One to Two to More Dimensions
Ernst's contributions to the field of NMR didn't end there. He returned to Switzerland and became the head of the NMR research group at ETH Zürich. In 1971, Ernst's first graduate student came back from summer school and told him about an interesting presentation by the Belgian scientist Jean Jeener, who had proposed a way of capturing more information by using a simple two-pulse sequence – in other words allowing the complex interactions within adjacent nuclei to evolve after the first radio pulse before hitting it with a second pulse. Jeener never developed his idea further, but using his original concept Ernst's lab successfully created a method known as two-dimensional NMR spectroscopy. This method worked by hitting compounds with radio pulses of varying lengths and intervals, creating a complex table of information that requires two Fourier transformations to convert into a NMR spectrum. This opened up the possibilities of NMR, allowing hopelessly difficult parts of NMR spectra to be analyzed, and making it possible to find out which atoms are closely linked to others in a molecule.
NMR could now advance to the stage at which it could be used to identify the structure of large biomolecules like proteins, which contain thousands of atoms. Kurt Wüthrich pioneered the NMR analysis of proteins through his inventions at the beginning of the 1980s, and this led to NMR becoming an important alternative to X-ray crystallography. NMR has the advantage that it can assess proteins in their natural state in solution, unlike crystallography, which requires the protein to be in a crystalline form. „Direct practical applications of biomacromolecular NMR in pharmaceutical industrial research include screening studies when a particularly important protein is being considered as a target for new drugs. The protein is exposed to a variety of small molecules, potential leads for a new drug. When a particular small molecule interacts with the macromolecule, the NMR spectrum of the macromolecule will change. If the resonances of the macromolecule have been assigned, it is also possible to determine which residues are involved in the interaction, and the potential use of the small molecule in further drug development can be assessed.“[8]
Wüthrich and Ernst partly worked together during the 1970s. To better understand the fast progress of NMR methods, it is interesting to see how each of them acclaims the achievements of his colleague from a different perspective:
(00:20:30 - 00:23:12)
(00:19:37 - 00:25:39)
From Molecules to Medical Diagnosis
Two-dimensional NMR spectroscopy could also be applied to imaging, and this paved the way to the invention of magnetic resonance imaging (MRI) for medical diagnosis. In the 1970s, the decade that witnessed the successful introduction of computer tomography, this invention was initiated and brought forward against the reluctance of both the scientific and the radiologic community with an enormous energy and persistence by Paul Lauterbur, a professor of chemistry at the State University of New York in Stony Brook, and facilitated in its technical applicability by Peter Mansfield, a professor of physics at the University of Nottingham. Two years after both scientists had shared the Nobel Prize in Physiology or Medicine, they both lectured in Lindau in 2005, Lauterbur on „From molecules to mice and back again” and Mansfield on “Real-time MRI: Echo-planar imaging”. Unfortunately, both presentations have not been recorded. To introduce the invention of MRI, we therefore rely again on Richard Ernst and Kurt Wüthrich and watch their brief descriptions of this ingenious technology:
(00:31:35 - 00:33:18)
(00:13:13 - 00:15:18)
MRI was introduced into practical medicine in the early 1980s. Initially, there were twelve machines worldwide, and everybody working with MR imaging knew each other. Today, approximately 25,000 MRI machines are in use worldwide. About 2,000 MR imaging units are sold worldwide every year. After an average of seven years, machines are being replaced.[9] Peter Lauterbur, however, did not get a patent on his basic discovery when he applied for it in the 1970s. Officials from his university declined his patent application, reasoning that the foreseeable applications of his discovery would not cover the expense of securing a patent.[10]
While MRI will continue to play a very important role in medical diagnosis, NMR is expected to deliver the more insights into nature’s structures and score, the deeper scientists dive into the dynamic processes within the nanocosmos of living cells and the less crystallography can help them there. Spectrometers will continue to increase in size and strength. At Frankfurt University, for example, where the NMR story began with the Stern-Gerlach experiment almost 100 years ago, one of the world’s first 1,2 Gigahertz NMR machines with a height of more than four meters and a weight of ten tons will be installed soon. It could enable researchers to study proteins and RNA in the cell at physiological concentrations, light activated states of membrane receptors at millisecond time-resolution or the formation of macromolecular complexes.
Footnotes:
[1] Amos Elon. The pity of it all. A portrait of the German-Jewish epoch 1743-1933. New York 2002, p. 362.
[2] Richard Feynman. Feynman lectures on Physics Complete Volumes 2, p. 445.
[3] Luisa Bonolis. Research Profile Otto Stern. With regard to NMR, Luisa Bonolis’ Research Profiles of Isidor Isaac Rabi, Felix Bloch and Richard Ernst within this mediatheque are also highly recommendable.
[4] Bretislav, F. and Herschbach, D. (2003) Stern and Gerlach: How a bad cigar helped reorient atomic physics. Physics Today 56: 53-59, p. 53.
[5] cf. http://www.magnet.fsu.edu/education/tutorials/pioneers/rabi.html
[6] cf. http://www.nobelprize.org/educational/physics/imaginglife/documents/article_che_1991.html
[7] Luisa Bonolis. Research Profile Richard Ernst.
[8] cf. http://www.nobelprize.org/nobel_prizes/chemistry/laureates/2002/advanced-chemistryprize2002.pdf, p. 10
[9] cf. Magnetic Resonance. A peer-reviewed, critical introduction http://www.magnetic-resonance.org/ch/21-01.html
[10] cf. http://www.nobelprize.org/educational/physics/imaginglife/documents/article_med_2003.html