X-ray crystallography
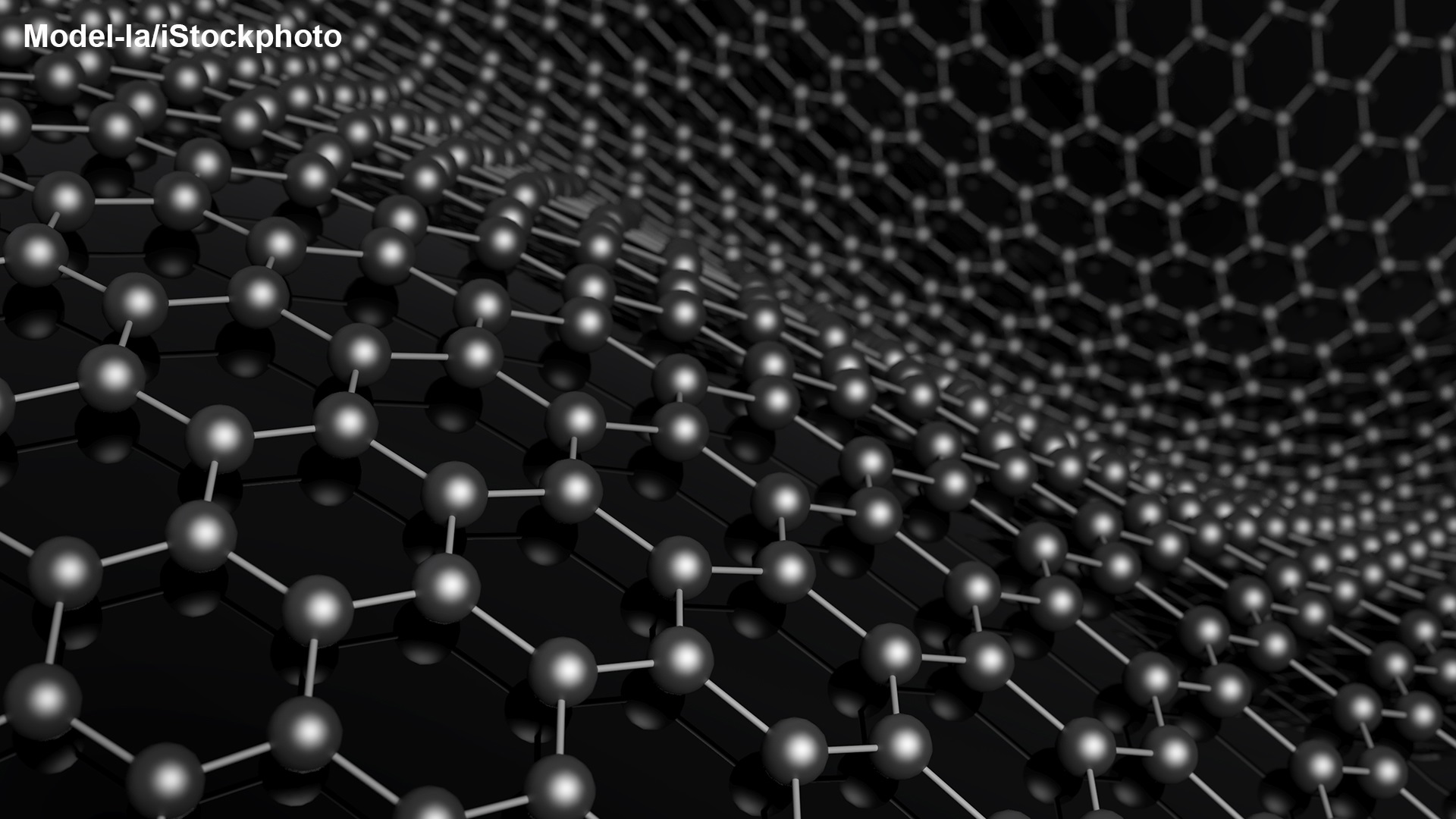
by Anders Bárány
Nobel Prizes and X-ray crystallography
In the autumn of 1895, Alfred Nobel must have been thinking a lot about the new (and final) version of his will, which was signed in the presence of witnesses in Paris on November 27. As is well known, Nobel mentioned physics as the first prize area to be rewarded and the physics Nobel Laureates always receive their prizes first. What Nobel could not be aware of in the autumn of 1895 was a research project carried out at the same time in Würzburg by the man who, in 1901, would receive the very first Nobel Prize in Physics and thus the very first Nobel Prize all categories. His name was Wilhelm Conrad Röntgen, and during an investigation of cathode rays he made a remarkable discovery already on the 8th of November. When the cathode rays, that we now know are energetic electrons, hit the wall of the evacuated glass tube that housed the electrical connections, a new kind of rays were emitted. In his first communication on the discovery, in December 1895, Röntgen named them X-rays, the “X” being used, as in mathematics, for something unknown. We now know that X-rays are energetic electromagnetic waves with a very short wavelength, today typically measured in terms of a nanometer, i.e. 10-9 meter. We all know that X-rays are very useful in practical medicine, since they penetrate most matter and allow insight into our bodies. But what will be described in this Topic Cluster is another use of them: Since the nanometer scale also happens to be the typical scale for the atomic and molecular structure of matter, X-rays have become the so-far most important tool for probing this structure.
Crystallography as such is a very old topic, at times involving quite a lot of mathematics. But X-ray crystallography starts in 1912 with a discovery by Max von Laue (Nobel Prize in Physics 1914) and collaborators. They found that crystals bend (diffract) X-rays into certain preferred directions. Since X-rays have the property of darkening photographic plates, putting such plates behind an irradiated crystal will produce photographs with spots. The spots form regular patterns, and the preferred directions can thus be stored on the photographic plates and analysed according to location and intensity. This diffraction effect was quickly explained as a wave phenomenon, an interference effect between X-ray waves reflected from different crystal planes, by Lawrence Bragg (Nobel Prize in Physics 1915). Together with his father William (with whom he shared the Nobel Prize) he used these so-called diffraction patterns to determine the structure of many simple crystals.
Since the pioneering work led by von Laue and the two Braggs, X-rays have become the dominant tool in crystallography, even though neutrons and (sometimes) electrons are also used. For a chemist having, e.g., synthesized a new molecule, the standard method to get at its precise structure is to get crystals of it and then use X-ray diffraction. A fairly large number of Nobel Prizes in Chemistry and some in Physiology or Medicine have rewarded work done using X-ray diffraction methods to unravel the structure of biologically important molecules, “the molecules of life”. To a large extent, this development started with a young mathematically inclined scientist from William Bragg’s laboratory in London, J.D. Bernal, who eventually moved to the physics institute in Cambridge, the Cavendish Laboratory, led by Ernest Rutherford (Nobel Prize in Chemistry 1908). In the 1920’s, when the scientific community realized that proteins are individual molecules and not aggregates of smaller molecules, Bernal had the idea that also these large molecules could be crystallized and analysed using X-ray diffraction. He started a project led by Max Perutz (Nobel Prize in Chemistry together with John Kendrew 1962) as well as one led by Dorothy Crowfoot Hodgkin (Nobel Prize in Chemistry 1964). Perutz and Hodgkin both performed heroic 20+ year long searches for the structure of proteins (haemoglobin and insulin, respectively). On the way these pioneers, who of course had no computers when they started their projects, had to overcome a large number of technical and fundamental problems and had to devise their own methods of investigation. While the projects of Perutz, Kendrew and Crowfoot Hodgkin mark the beginning of molecular biology, meanwhile the molecular genetics revolution was started by the intelligent modelling of the structure of the nucleic acid (DNA) by Francis Crick and James Watson, also at the Cavendish Laboratory, using X-ray diffraction data taken by Maurice Wilkins and Rosalind Franklin in London (Nobel Prize in Physiology or Medicine 1962 to Crick, Watson and Wilkins). What came out of these projects was not only the atomic structure of the proteins and the DNA, respectively, but also that the structure could explain some of the reactions that the molecules take part in. This has become the overriding motivation for all the later studies of the structure of proteins: How do they behave “at work”.
Since the 1950’s, computers have become important tools used to disentangle the structure of more and more complex proteins with the primary data collected from X-ray diffraction. Today the X-rays are usually produced in high-energy synchrotron storage rings, where electrons or positrons emit X-rays when they are accelerated in the bending magnets or in different types of magnetic insertion devices. At the X-ray beam lines, research equipment is put up by university scientists, but also by companies performing structure determination on a commercial basis. Examples of successful investigators rewarded with Nobel Prizes are Johann Deisenhofer, Robert Huber and Hartmut Michel, who determined the structure and function of a two-dimensional so-called membrane protein, a photosynthetic reaction centre (Nobel Prize in Chemistry 1988); John Walker, who determined the structure and dynamics of the ATP molecule, which surprisingly contains a rotating part (Nobel Prize in Chemistry 1997 with Paul Boyer); Roderick MacKinnon, who unravelled the structure and dynamics of the ion channel protein, which acts as a tunnel into the nerve cells (one of the Nobel Prizes in Chemistry 2003); Roger Kornberg, who determined the molecular mechanism that copies single strands of DNA into messenger RNA (Nobel Prize in Chemistry 2006); Venkatraman Ramakrishnan, Thomas Steitz and Ada Yonath, who managed to determine the structure and function of the protein factory of the cells, the ribosome molecule that gets its information from the messenger RNA (Nobel Prize in Chemistry 2009); Brian Kobilka and Robert Lefkowitz, who as a part of their investigation determined the structure of G protein-coupled receptors using X-ray crystallography (Nobel Prize in Chemistry 2012).
But even though the actual X-ray diffraction work has become more and more of a standard procedure, the problem of producing and keeping complicated protein crystals intact during the investigations is still something that has to be solved over and over again. As if this problem was not enough, there is also a more fundamental one, the so-called phase problem. Without going into details, the phase problem is connected with the fact that the spots on a photographic plate can give you a measure of the intensity of an electromagnetic wave, but not of its phase. Since the latter is needed for a mathematically stringent extraction of the crystal structure from the diffraction data, a number of practical methods have been used to overcome this problem. One is producing crystals with and without insertions of heavy atoms and comparing diffraction data for the two kinds of crystals. But in the 1950’s, the mathematician Herbert Hauptman and the physical chemist Jerome Karle devised a probabilistic approach to the problem and showed that by solving a certain large set of equations, the crystal structure can in fact be determined in a direct method (Nobel Prize in Chemistry 1985).
Lindau Lectures and X-ray crystallography
In the Lindau Mediatheque database of lectures that have been recorded since 1951, there are today (2013) around 50 lectures by Nobel Laureates who have used X-ray crystallography as a method of investigation. Instead of following a purely historic path among the lectures, we here jump immediately to one of the more recent ones. This lecture describes how one of the most complex protein structures, the ribosome, has been revealed using this method. The ribosome is the “factory” molecule that produces proteins as ordered by the genetic code through the messenger RNA. The first snippet comes from a lecture given in 2011 by one of the 2009 Nobel Laureates in Chemistry, Ada Yonath. She describes, in particular, how she was led to the idea that one could produce crystals of such a complicated molecule as the ribosome and how she and her collaborators overcame the first set of difficulties in using these crystals to unravel the structure of the molecule. The title of the talk is “Climbing the Everest Beyond the Everest”.
(00:29:45 - 00:37:22)
Next we go back to the intellectual discoverer of X-ray diffraction, Max von Laue, who lectured on “X-ray Interferences” at the very first physics meeting in Lindau, in 1953. This historic lecture snippet is in German, which of course by far was the dominating science language at the time von Laue received his Nobel Prize. Even though the year of the prize officially is 1914, he did not actually receive it until 1920. That year the Nobel Foundation arranged two Nobel Ceremonies, one in June for the Laureates who had not been able to come to Stockholm because of WWI and one in December (as usual) for the Laureates of the year. This explains why von Laue in the beginning of his talk refers to his visit to Stockholm 33 years ago! In the lecture snippet, he talks about the discovery made in Munich in 1912. Of special interest is the role of Arnold Sommerfeld, who was the head of the department and who suggested the investigation, and of the two experimental physicists W. Friedrich and P. Knipping, who performed the actual measurements. If given today, the Nobel Prize could very well have included also one or two of these scientists (but not all three!).
(00:08:08 - 00:16:05)
Luckily enough, also 1915 Physics Nobel Laureate Lawrence Bragg managed to come to Lindau once, in 1968, to give a lecture from which the next snippet is taken. When Bragg, at the end of the 1930’s, took over the directorship of the Cavendish Laboratory from Ernest Rutherford, there was already an ongoing investigation there of molecules of life using X-ray diffraction. The investigator was Max Perutz, who studied haemoglobin. He was eventually joined by John Kendrew, who looked for the structure of myoglobin. Another investigation was later planned for nucleic acid (DNA) with Francis Crick as investigator and with James Watson about to join. But even though these projects in a sense were quite unphysical, the physicist Bragg kept an open mind and let them go on in his laboratory. So with the Nobel Prize outcome of the investigations, Bragg could say at the beginning of his talk that he represented not only his own subject physics, but also chemistry and physiology/medicine, and therefore ought to be invited every year! The title of his talk is “History of the Determination of Protein Structure”.
(00:01:35 - 00:08:40)
As described above, already in the 1930’s, the brilliant scientist J.D. Bernal had conceived the idea of using X-ray crystallography to determine the structure of proteins. His disciple Dorothy Crowfoot Hodgkin went to Oxford and started work on the insulin molecule in her laboratory. It took her about 20 years to determine the structure, but on the way she also determined other structures such as vitamin B12 and penicillin and received the 1964 Nobel Prize in Chemistry before she was actually finished with insulin. She obviously fell in love with the Lindau meetings and lectured there many times. Here we present a snippet of her 1980 lecture entitled “History and the X-ray Analysis of Protein Crystals”, in which she tells the story of the beginnings of the crystallographic work on proteins.
(00:12:28 - 00:20:15)
As already noted, on a fundamental mathematical level, it is really impossible to determine the structure of any crystal or large molecule by only using X-ray diffraction as described above. The reason is the phase problem, where the term “phase” refers to the wave property of X-rays. Even though experimental tricks to overcome this problem have been developed, the 1985 Nobel Prize in Chemistry was given for a theoretical solution to the problem. Around 1950, Jerome Karle and Herbert Hauptman devised the so-called direct method, which turned out to be very useful when computers became available. Both have come frequently to Lindau and here we present a snippet from Hauptman’s very instructive 1989 lecture “A New Minimal Principle in X-ray Crystallography”.
(00:03:09 - 00:11:11)
As pointed out several times above, computers have become extremely powerful tools for the X-ray diffraction method and some parts of the investigations have almost become routine. But for large biological molecules, there is also the problem of making high-quality crystals. In 1988, Johann Deisenhofer, Robert Huber and Hartmut Michel received the Nobel Prize in Chemistry for determining the structure and function of a so-called membrane protein, a protein no one had thought could be crystallized. With their German connection, the three Nobel Laureates are invited to Lindau every year and they have responded to this invitation several times. Here we present a snippet from Hartmut Michel’s 1998 lecture “From Photosynthesis to Respiration: Structure and Function of Energy Transforming Membrane Protein Complexes”, in which he shortly describes a very interesting high-tech approach to getting useful crystals.
(00:13:00 - 00:15:55)
Another complicated protein molecule that no one believed could be crystallized is the so-called ion channel. It was shown to be amendable to X-ray diffraction analysis by a young electro chemist, Roderick MacKinnon. The ion channel sits on the surface of nerve cells and acts as a gate-keeper, only letting particular ions through. This is the way that electrical signals are transmitted along the nerves, which was known earlier, but the way the gate-keeper actually works was unknown. A snippet from a lecture given in 2005 by one of the 2003 Nobel Laureates in Chemistry, Roderick MacKinnon, is presented here. The title of the talk is “Ion Channels: Life’s Electronic Hardware”.
(00:15:06 - 00:19:01)
Finally, to end up where we started, here is a snippet from one of Ada Yonath’s co-recipients of the Nobel Prize in Chemistry 2009, Thomas Steitz, who also lectured at Lindau the same year as she, in 2011. Their molecule, the ribosome, is the same, but the lecture entitled “From the Structure of the Ribosome to the Design of New Antibiotics” is quite different. Knowledge of the structure of bacterial ribosome can help design new antibiotics that hinder the ribosomes “at work”.
(00:03:20 - 00:10:20)
Additional Lectures by the Nobel Laureates associated with X-ray crystallography
Introductory Mini Lecture on X-ray crystallography.
Max von Laue 1956: "From Copernicus to Einstein".
James Watson 1967: "RNA Viruses and Protein Synthesis".
Dorothy Crowfoot Hodgkin 1970: "Structure of Insulin".
Dorothy Crowfoot Hodgkin 1983: "Insulin 1983".
Max Perutz 1986: "Hemoglobin as Receptor for Drugs: Stereochemistry of Bonding".
Maurice Wilkins 1987: "Ideals of Science and Medicine".
Dorothy Crowfoot Hodgkin 1989: "A Life in Science".
Johann Deisenhofer 1989: "The Three-Dimensional Structure of a Membrane Protein".
Hartmut Michel 1989: "Structure and Function of a Biological Light Energy Converter".
Johann Deisenhofer 2002: "Back to Proteins".
Johann Deisenhofer 2006: "Structural Insights into Cholesterol Homeostasis".
Jerome Karle 2006: "Kernel Energy Methods Illustrated with Peptides".
Robert Huber 2007: "Proteolysis and its Regulation, a Molecular Basis".
Johann Deisenhofer 2008: "Structural Biology – Quo Vadis?".
Robert Huber 2009: "Molecular Machines for Protein Degradation Inside Cells".
Robert Huber 2010: "Basic Science and Co-entrepreneurship, My Experience".
Ada Yonath 2010: "The Amazing Ribosome".
Robert Huber 2011: "Proteasome and DegP Protease, Mechanisms and Drug Design".
Robert Huber 2013: "Proteases and Their Control in Health and Disease".
Brian Kobilka 2013: "G Protein Coupled Receptors: Challenges for Drug Discovery".
Ada Yonath 2013: "Curiosity and its Fruits: From Basic Science to Advanced Medicine".